Kodiak 3D Lidar
optics
Kodiak 3D Lidar (GSC-TOPS-212)
Provides low cost and highly reliable 3D lidar images in nearly any lighting condition.
Overview
High orbit satellite servicing and planetary science missions are presented with unique challenges, including a harsh environment and long mission life, coupled with the need for long range and high speed and accurate measurements. Conventional systems suffer from high costs of bringing other solutions to market and questionable reliability.
The Technology
NASA Goddard Space Flight Center has developed a 3D lidar system that consists of microelectromechanical systems (MEMS) beam steering, high performance reconfigurable computing, and an in-depth understanding of systems level integration. Kodiak combines a 3D MEMS scanning lidar with a long range narrow FOV telescope to produce a flexible and capable space flight ranging system. Also included is SpaceCube-level processing power to host a variety of algorithms enabling sensing and 6 degrees of freedom.
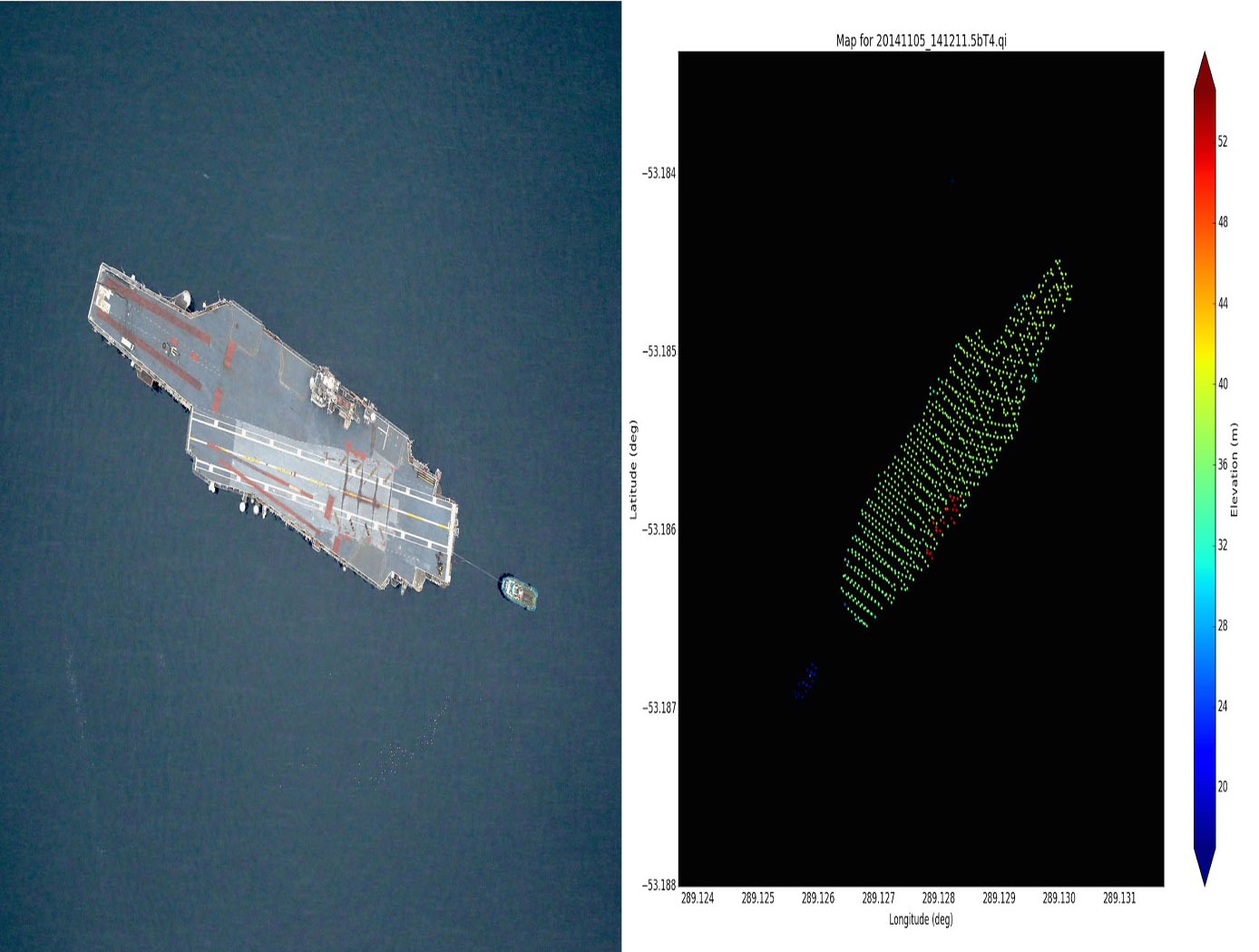
Benefits
- Lower cost
- Higher performance and reliability
- Reconfigurable
- Low SWaP
- Capable of generating 3D imaging in nearly any lighting condition from long ranges
Applications
- Space detection and ranging
- Proximity laser ranging
- Autonomous vehicles
Technology Details
optics
GSC-TOPS-212
GSC-18054-1
Similar Results

Goddard's Reconfigurable Laser Ranger (GRLR)
NASA Goddard Space Flight Center has developed a low cost, modular, and flexible space flight laser range finder consisting of optics, electronics, and interfaces for satellite servicing missions (i.e. Restore-L) using customized optics. Built upon previous NASA technologies, the system also consists of a high dynamic range receiver and adjustable laser for a wide range of measurements (i.e. multiples of km to sub-meter).

SmallSat Common Electronics Board (SCEB) Complement Board Design: Memory Card
The innovation is a miniaturized memory board that will have up to 96 GB of NAND Flash memory along with either a radiation tolerant FPGA or a set of three commercial FPGAs. The memory board is designed to interface with the standard subsystems of Goddards Modular SmallSat Architecture (GMSA). While previous memory cards are larger, this one is designed to fit within a 1U form factor.

Method and Associated Apparatus for Capturing, Servicing, and De-Orbiting Earth Satellites Using Robotics
This method begins with the optical seeking and ranging of a target satellite using LiDAR. Upon approach, the tumble rate of the target satellite is measured and matched by the approaching spacecraft. As rendezvous occurs the spacecraft deploys a robotic grappling arm or berthing pins to provide a secure attachment to the satellite. A series of robotic arms perform servicing autonomously, either executing a pre-programmed sequence of instructions or a sequence generated by Artificial Intelligence (AI) logic onboard the robot. Should it become necessary or desirable, a remote operator maintains the ability to abort an instruction or utilize a built-in override to teleoperate the robot.

Automata Learning in Generation of Scenario-Based Requirements in System Development
In addition, the higher the level of abstraction that developers can work from, as is afforded through the use of scenarios to describe system behavior, the less likely that a mismatch will occur between requirements and implementation and the more likely that the system can be validated. Working from a higher level of abstraction also provides that errors in the system are more easily caught, since developers can more easily see the big picture of the system.
This technology is a technique for fully tractable code generation from requirements, which has an application in other areas such as generation and verification of scripts and procedures, generation and verification of policies for autonomic systems, and may have future applications in the areas of security and software safety. The approach accepts requirements expressed as a set of scenarios and converts them to a process based description. The more complete the set of scenarios, the better the quality of the process based description that is generated. The proposed technology using automata learning to generate possible additional scenarios can be useful in completing the description of the requirements.

Miniaturized Astrometric Alignment Sensor
The Miniaturized Astrometric Alignment Sensor advances satellite capabilities for astrophysical measurements, necessary for formation flying, relative navigation, and virtual telescope capabilities. The sensor is a single assembly consisting of a small, low powered camera assembly. The sensor detects stellar objects from which both stellar and object tracking are performed. The sensors components consist of a low power camera assembly, interchangeable lenses, camera power supply, and image processing software and algorithms. The system functions by searching and identifying objects in the camera's field of view and tracking the objects against a selected star pattern with a central body of interest in the sensor's field of view.
The Miniaturized Astrometric Alignment Sensor makes it possible to measure a spacecrafts altitude and orientation with respect to known stellar objects. The instrument takes an image of a patch of sky, identifies the stars in that field of view, and compares the field view with a stored star map. The data is processed with a dedicated processor attached to the instrument to spell out the attitude and orientation of a spacecraft.