Durable Polyimide Aerogels
materials and coatings
Durable Polyimide Aerogels (LEW-TOPS-133)
Stronger and flexible, polyimide aerogels provide insulation and structural support
Overview
Innovators at NASA's Glenn Research Center have developed cutting-edge polyimide aerogel technologies that are 500 times stronger than traditional silica aerogels. These durable polyimide aerogels are highly flexible and foldable in contrast to traditional silica aerogels, which shed dust particles and are notoriously fragile and brittle. Polyimide aerogels possess remarkable strength, flexibility, and moisture resistance and make excellent "wrappable" insulators. No other aerogel possesses the compressive and tensile strength of this organic polyimide aerogel while still retaining its ability to be flexibly folded to contour to whatever shape is needed such as automotive shields, pipeline insulation, and protective clothing. Glenn's durable polyimide aerogels combine the robust nature of a polyimide network with low thermal and sound conductivity. This unique combination of strength, flexibility, and exceptional insulating properties make these aerogels ideal for insulation (thermal, electrical, and acoustic), structural elements and more - at a fraction of the weight of similar materials.
The Technology
Aerogels are highly porous, low-density solids with extremely small pore sizes, fabricated by forming a gel from a solution in the wet-gel state that is then converted to the dry-solid state without compaction of the porous architecture. Aerogels make excellent electrical, thermal, and acoustic insulators. However, most inorganic silica aerogels are fragile and shed dust. The NASA Glenn team is the first to synthesize three-dimensional polymer aerogel networks of polyimides cross-linked with multifunctional amine monomers. Compared to silica aerogels, these aerogels retain small pore sizes and low thermal conductivities, but are distinguished by their flexibility. Polyimide aerogels are not brittle, fragile, or dusty like silica aerogels. Plus, polyimide aerogels possess the beneficial characteristics and strength of polyimide materials. The results are cross-linked polyimide aerogels with little shrinkage, low densities, high compression and tensile strengths, and good moisture resistance. They can be fabricated or machined into net shape parts, which are strong and stiff, or cast as thin flexible films with good tensile properties. Extremely customizable, polyimide aerogels can be formed into any configuration (e.g., wrapped around a pipe, sewn into protective clothing, or molded into a panel to act as a heat shield in a car). In short, Glenn's innovation improves the performance, adaptability, and affordability of aerogels in a broad number of applications.
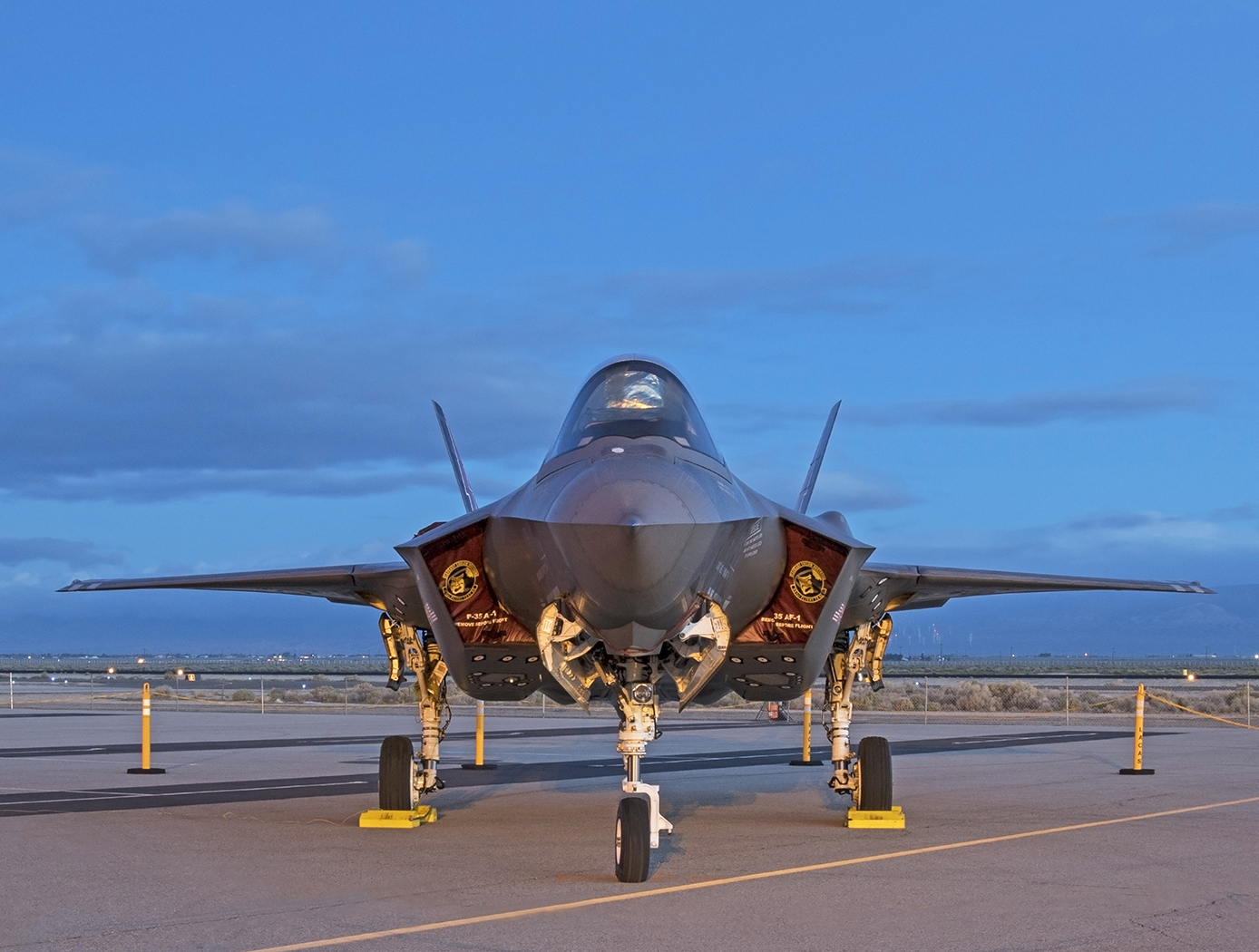
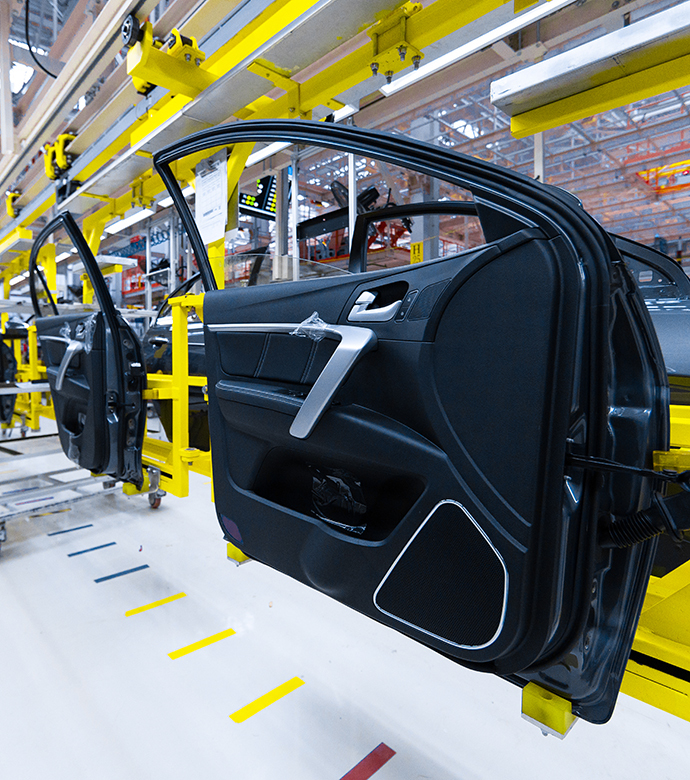
Benefits
- Strong: 500 times stronger than traditional silica aerogels
- Low thermal conductivity: 2 to 10 times improved performance over polymer foam insulation
- Easy to produce: Simple fabrication techniques enable lower costs
- Flexible and lightweight: Can be crafted into thin, lightweight films (as thin as 0.5 mm) and "wrappable" insulation
- Robust: Can retain physical and mechanical properties in thermally and chemically demanding environments
Applications
- Aerospace
- Antennas
- Automotive
- Biotech (e.g., tissue engineering scaffolds)
- Chemical manufacturing (e.g., catalytic supports)
- Communications (e.g., wave guides, wire cladding)
- Filters
- High-performance sports
- Insulation
- Power (e.g., battery separators, capacitors)
- Protective gear
- Satellites
- Sensors
- Unmanned vehicles
Technology Details
materials and coatings
LEW-TOPS-133
LEW-18486-2
LEW-18825-1
LEW-18864-1
LEW-19108-1
LEW-19200-1
LEW-19200-2
LEW-19230-1
LEW-19230-2
LEW-19421-1
LEW-18893-1
LEW-18893-2
LEW-18825-2
Similar Results

Optically Transparent Polyimide Aerogels
Innovators at NASA Glenn have devised a new method for harnessing the high transmission and clarity associated with optical glasses in a robust polyimide aerogel. This process uses sol-gel synthesis technology with aromatic dianhydrides and diamines as the precursors, and a trifunctional triacid chloride that arranges itself into a three-dimensional (3D) matrix with a low refractive index. The liquid portion of the gel is then removed by supercritical fluid extraction in order to produce the polyimide aerogel and maintain the desired 3D structure without pore collapse. The result is a cross-linked polyimide aerogel that allows for light wave transmittance while retaining low thermal conductivity. This unique material can be made into thin blocks, or highly flexible films as thin as 0.5 mm. While some embodiments have a yellow color, other embodiments may be nearly colorless. When compared to high-opacity polyimide aerogels, they have much greater surface area (up to 880 m2/g) and a very homogenous pore size (10 to 20 nm) with only a minor penalty in density (0.15 g/cc vs 0.10 g/cc). These strong, optically transparent aerogels incorporate a number of unique properties with applicability to a host of potential new applications, making this innovation a game-changer in the global aerogel market. Glenn welcomes co-development opportunities.

Polymer Cross-Linked Aerogels (X-Aerogels)
Researchers at NASA's Glenn Research Center have developed an approach to significantly improve the mechanical properties and durability of aerogels without adversely affecting their desirable properties. This approach involves coating conformally and cross-linking the individual skeletal aerogel nanoparticles with engineering polymers such as isocyanates, epoxies, polyimides, and polystyrene. The mechanism of cross-linking has been carefully investigated and is made possible by two reactions: a reaction between the cross-linker and the surface of the aerogel framework and a reaction propagated by the cross-linker with itself. By tailoring the aerogel surface chemistry, Glenn's approach accommodates a variety of different polymer cross-linkers, including isocyanates, acrylates, epoxies, polyimides, and polystyreneenabling customization for specific mission requirements. For example, polystyrene cross-linked aerogels are extremely hydrophobic, while polyimide versions can be used at higher temperatures. Recent work has led to the development of strong aerogels with better elastic properties, maintaining their shape even after repeated compression cycling. By tailoring the internal structure of the silica gels in combination with a polymer conformal coating, the aerogels may be dried at the ambient condition without supercritical fluid extraction.

Polyamide Aerogels
Polyamides are polymers that are similar to polyimides (another polymer that has been developed for use in aerogels). However, because the amide link is a single chain while the imide link is a ring structure, polyamide aerogels can be made less stiff than polyimides, even though a similar fabrication process is used. The precursor materials can be made from any combination of diamine and diacid chloride. Furthermore, NASA Glenn researchers have found methods for using combinations of diamines and disecondary amines to produce polyamide aerogels with tunable glass transition temperatures, for greater control of features such as flexibility or water-resistance.
In the first step of the fabrication process, an oligomeric solution is produced that is stable and can be prepared and stored indefinitely as stock solutions prior to cross-linking. This unique feature allows for the preparation and transport of tailor-made polyamide solutions, which can later be turned into gels via the addition of a small amount of cross-linker. When the cross-linking agent is added, the solution can be cast in a variety of forms such as thin films and monoliths. To remove the solvent, one or more solvent exchanges can be performed, and then the gel is subjected to supercritical drying to form a polyamide aerogel. NASA Glenn's polyamide aerogels can be fully integrated with the fabrication techniques and products of polyimide aerogel fabrication, so hybrid materials which have the properties of both classes are easily prepared. As the first aerogels to be composed of cross-linked polyamides, these materials combine flexibility and transparency in a way that sets them apart from all other polymeric aerogels.

Aerogel Reinforced Composites
GRC's aluminosilicate aerogel composites are fabricated using a sol-gel technique. A sol is formed by hydrolyzing an alumina dispersion in acid solution; the alumina may be combined with a silicon precursor to create a sol.
Fabrics, papers, and felts are used as reinforcing fibers to form an aerogel composite. The aerogel adheres to the reinforcement without use of sizing or organic binders. (In the case of sized fabrics, the sizing is first removed by heat cleaning.) Composites can be fabricated in a batch process, impregnating individual layers of paper, felt or fabric with the precursor sol, or in a roll-to-roll process. The sol is allowed to gel, and then aged for several days prior to supercritical drying using liquid CO2. Heat treatment of the super critically dried composites can be used to tailor the alumina or Aluminosilicate crystal structure and pore size.
In contrast to commercially available insulations, GRC's innovation provides extremely low thermal conductivity (60 mW/m-K at 900°C in argon) at high temperatures, thus enabling use at higher temperatures and improving applicability. In addition, GRC's unique process provides very good adhesion of the aerogel to its reinforcing fibers in alumina papers and zirconia felts, eliminating the spalling seen in other aerogel composites. Finally, GRC's innovation demonstrates low density and extreme resilience to high temperatures and harsh conditions. Seven layers of composite material of 1.25 mm/layer produced a temperature drop of 700°C when tested in the 8-foot high-temperature wind tunnel (8 HTT) at NASA's Langley Research Center. The technology also has withstood heat tests of up to 1200°C. In combination with other insulators, it has withstood fluxes of up to 65 W/cm2, producing a temperature drop of 625°C across 8 mm.

Self-Healing Wire Insulation
Insulation is necessary on electrical wires in order to protect electrical systems from shorting. In high voltage systems such shorting can lead to sparking and fires. Many lives have been lost due to electrical wire insulation failure. Many man hours are also expended in the repair and inspection of electrical wiring in order to attempt to prevent wire failure. Wire insulation with a built in "self-healing" capability would greatly improve the safety of systems containing electrical wiring. Such insulation would require far less inspection and repair time over the lifetime of the system.
Polyimides such as Kapton are an integral part of high performance electrical wire insulation. Traditional polyimides are very inert to solvents and do not melt. A new set of polyimides, developed for use as films for the manual repair of high performance electrical wire insulation, have a low melting point and can be dissolved in special solvents. These properties can be taken advantage of in self-healing polyimide films. Microcapsules containing a solvent soluble polyimide are prepared using industry standard inter-facial or in situ polymerization techniques. These capsules are then incorporated into a low melt polyimide film for use as either a primary electrical wire insulation or as one of several layers of a composite wire insulation. The low melt polyimide film substrate in which the microcapsules are incorporated has good solubility with the solvent used to dissolve the polyimide which makes up the fluid inside the microcapsule. Such a capsule filled insulation, when cut or otherwise damaged, will result in the release of the capsule contents into the cut or damage area. The solvent then dissolves a small amount of the surrounding polyimide insulation but will also begin the process of evaporation. The combination of these two processes allows for excellent intermingling of the healant and the surrounding substrate, resulting in a repair with superior bonding and physical properties.