Search
PATENT PORTFOLIO
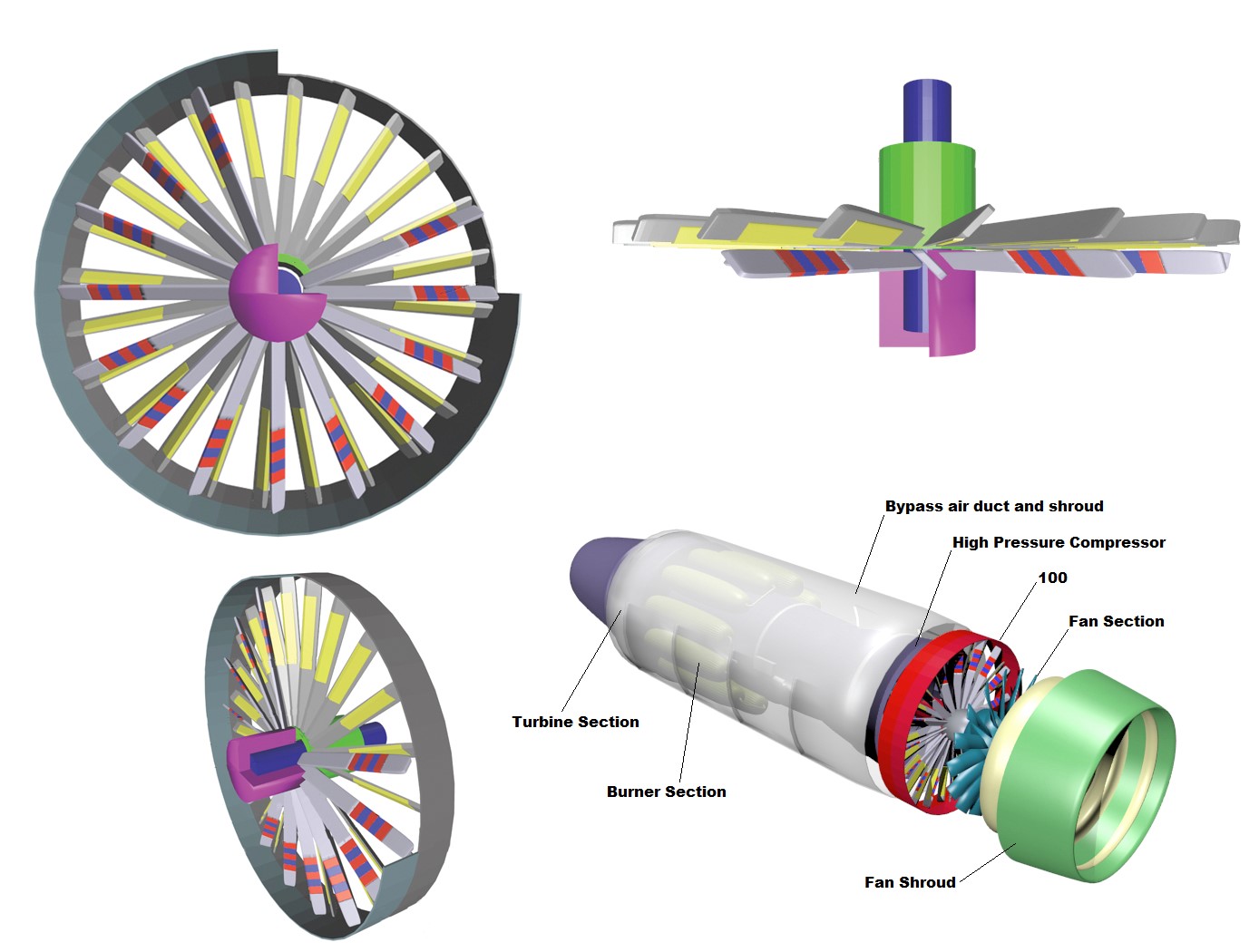
Axial Magnetic Flux Airflow Integrated Compressor-Generator-Motor Turbojet
The innovation uses the rotating blades of the compressor section to act as structural support for the generator. Since the compressor is the coolest part of the engine, it will reduce the potential for interference with magnetics and associated curie points of the permanent magnets. The placement of the generator in the cooler part of the engine flowpath (fan or compressor) will also improve the electrical insulation system's degradation and serve to improve overall system lifetime.
The configuration proposed by Armstrong's design would be an axial magnetic flux permanent magnet generator or motor. The electrical/mechanical interface could serve to deliver power to the shaft of the turbojet/fan or extract power from the shaft.
This axial electromagnetic flux design is more efficient for the combined function of aero-thermal heat transfer and generation of electricity. This is due to the relative amount of available cooling surface area, which has an advantage over radial designs given the total system volumetric aspect ratio of the generator/compressor section. When the system is viewed as a thermodynamic cycle, it is more efficient because it is essentially a regenerative cycle, with the heat of generation being fed back into the cycle instead of being released into the ambient surroundings
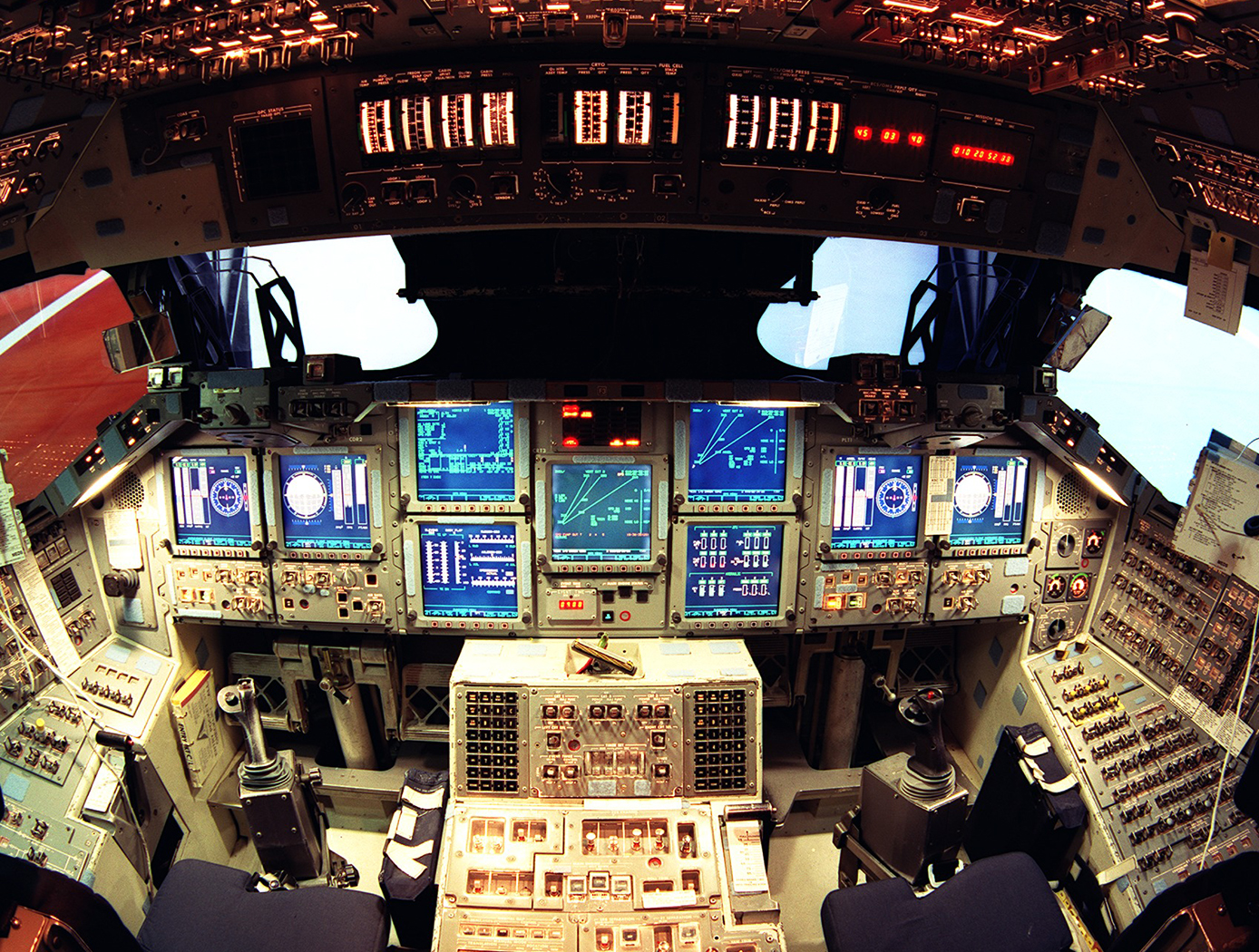
Sensing Magnetic Fields
This technology is part of Armstrong's portfolio of fiber optic sensing technologies known as FOSS. The innovation leverages Armstrong's cutting edge work in this area, including its patented FBG interrogation system, which allows for a diverse set of engineering measurements in a single compact system. In addition to magnetic field, other measurements include structural shape and buckling modes, external loads, and cryogenic liquid level. The system and measurement technology is commercially available for research applications. In addition to capitalizing on the significant advancements in fiber optic and laser technologies that have been made to support the telecommunications industry, Armstrong has also partnered with UCLA's Active Materials Lab (AML) to tap their expertise in the field of magnetics.
For more information about the full portfolio of FOSS technologies, see DRC-TOPS-37 or visit https://technology-afrc.ndc.nasa.gov/featurestory/fiber-optic-sensing
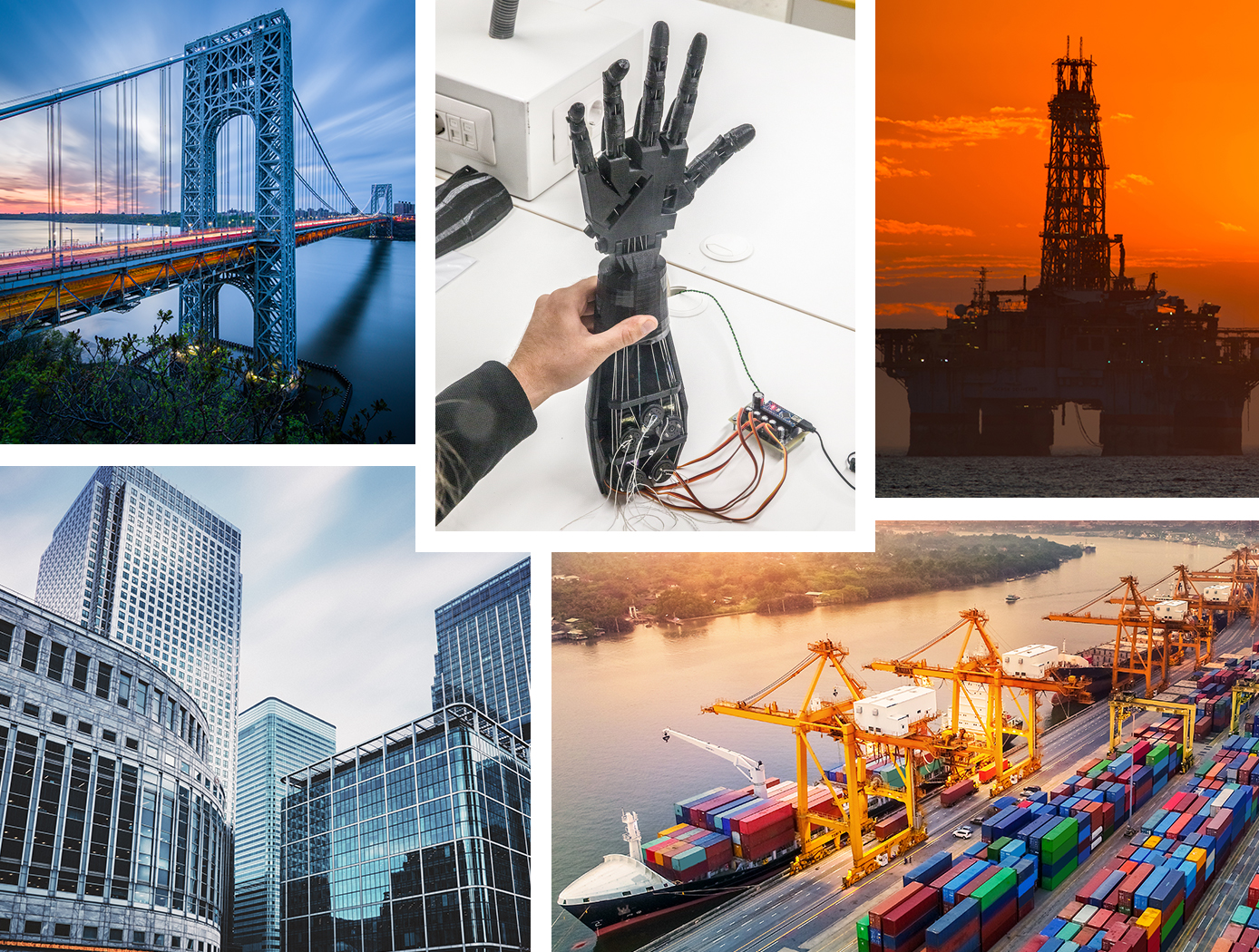
Adaptive Spatial Resolution Enables Focused Fiber Optic Sensing
This technology can be applied to most optical frequency domain reflectometry (OFDR) fiber optic strain sensing systems. It is particularly well suited to Armstrong's FOSS technology, which uses efficient algorithms to determine from strain data in real time a variety of critical parameters, including twist and other structural shape deformations, temperature, pressure, liquid level, and operational loads.
How It Works
This technology enables smart-sensing techniques that adjust parameters as needed in real time so that only the necessary amount of data is acquired—no more, no less.
Traditional signal processing in fiber optic strain sensing systems is based on fast Fourier transform (FFT), which has two key limitations. First, FFT requires having analysis sections that are equal in length along the whole fiber. Second, if high resolution is required along one portion of the fiber, FFT processes the whole fiber at that resolution. Armstrong's adaptive spatial resolution innovation makes it possible to efficiently break up the length of the fiber into analysis sections that vary in length. It also allows the user to measure data from only a portion of the fiber. If high resolution is required along one section of fiber, only that portion is processed at high resolution, and the rest of the fiber can be processed at the lower resolution.
Why It Is Better
To quantify this innovation's advantages, this new adaptive method requires only a small fraction of the calculations needed to provide additional resolution compared to FFT (i.e., thousands versus millions of additional calculations). This innovation provides faster signal processing and precision measurement only where it is needed, saving time and resources. The technology also lends itself well to long-term bandwidth-limited monitoring systems that experience few variations but could be vulnerable as anomalies occur.
More importantly, Armstrong's adaptive algorithm enhances safety, because it automatically adjusts the resolution of sensing based on real-time data. For example, when strain on a wing increases during flight, the software automatically increases the resolution on the strained part of the fiber. Similarly, as bridges and wind turbine blades undergo stress during big storms, this algorithm could automatically adjust the spatial resolution to collect more data and quickly identify potentially catastrophic failures.
This innovation greatly improves the flexibility of fiber optic strain sensing systems, which provide valuable time and cost savings to a range of applications.
For more information about the full portfolio of FOSS technologies, see DRC-TOPS-37 or visit https://technology-afrc.ndc.nasa.gov/featurestory/fiber-optic-sensing
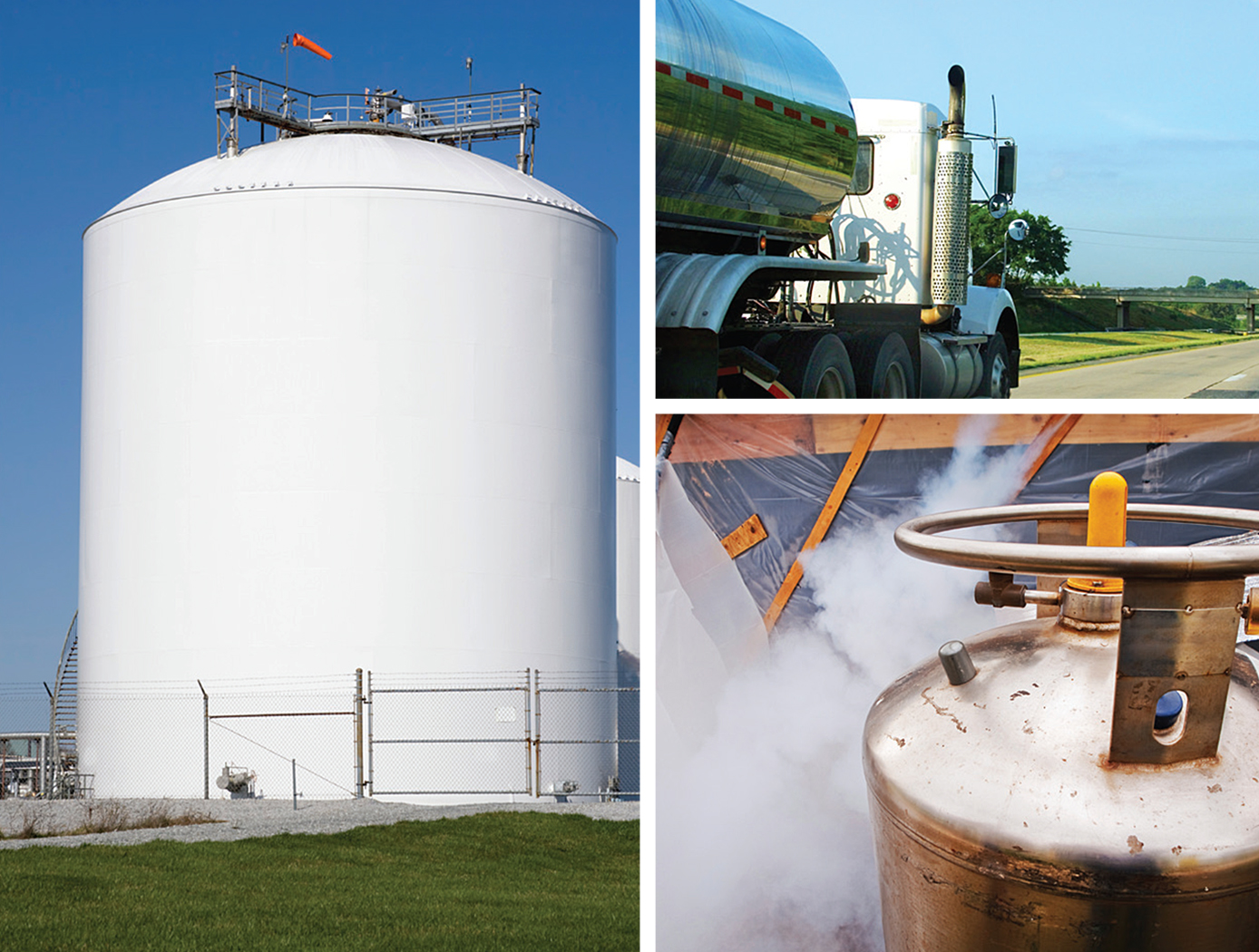
Streamlined Liquid Level Sensing Using Fiber Optics
Armstrong has developed a robust fiber optic–based sensing technology that offers extraordinary accuracy in liquid level measurements. The sensing system uses fiber optic Bragg sensors located along a single fiber optic cable. These sensors actively discern between the liquid and gas states along a continuous fiber and can accurately pinpoint the liquid level.
How It Works
The technology uses a resistive heater wire bundled with the optical fiber. The heater is pulsed to induce a local temperature change along the fiber, and the fiber Bragg grating data is used to monitor the subsequent cooling of the fiber. The length of fiber in the liquid cools more rapidly than the portion of the fiber in the gas above the liquid. The measurement system accurately establishes the location of this transition to within 1/4-inch.
Why It Is Better
Armstrong's liquid level sensing technology was originally developed to measure cryogenic liquid levels in rockets, and it represents a significant advancement in the state of the art in this application. Conventional methods for measuring cryogenic liquid levels rely on cryogenic diodes strategically placed along a rod or rack. The diodes are mounted in pre-selected, relatively widely spaced positions along the length of a rod; this configuration provides limited, imprecise data. Furthermore, each diode on the rod has two wires associated with it, which means a single system may require a large number of wires, making installation, connectivity, and instrumentation cumbersome.
Armstrong's novel technology provides liquid measurements with much greater precision, achieving measurements at 1/4-inch intervals. Furthermore, the streamlined system uses just two wires, which greatly simplifies installation and instrumentation. Due to its extraordinary accuracy and ease of use, Armstrong's measurement system offers important advantages for a wide range of applications beyond cryogenic liquids.
In Addition
Researchers have developed a new manufacturing process that improves the ability of fiber optic sensing systems to measure temperature and liquid levels when operating in humid environments. The process involves eliminating moisture from the optical fiber coating, then completing the sensor assembly within humidity-controlled conditions. The resulting sensor hardware provides precise and accurate measurements even when operating in a humid environment.
For more information about the full portfolio of FOSS technologies, see DRC-TOPS-37 or visit https://technology-afrc.ndc.nasa.gov/featurestory/fiber-optic-sensing
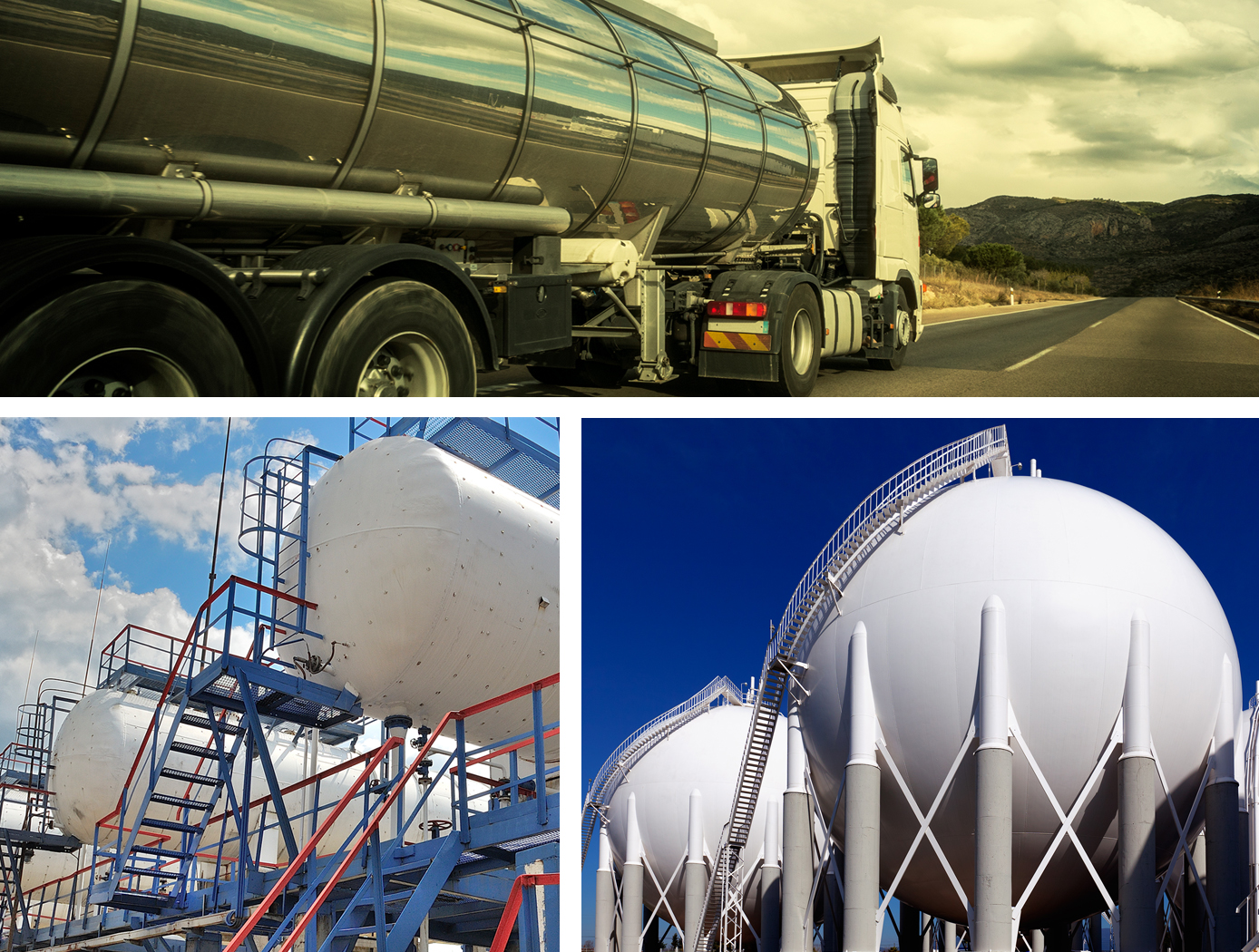
Enhanced Fabrication Improves Temperature Sensing in Cryogenic Humid Environments
This technology was developed to improve Armstrong's multi-patented FOSS system, which has long been used to measure temperature and liquid levels in cryogenic environments. When the sensing system's fibers trapped humidity from the surrounding environment before their submersion into cryogenic liquids, the moisture adversely affected outputs. A new manufacturing process solves this problem, increasing reliability and accuracy not only of NASA's FOSS but also any fiber optic sensing system.
How It Works
Armstrong has developed a two-step process to assemble the sensors. First, the bare sensor fiber is inserted into an oven to expel all moisture from the fiber coating. Then, the moisture-free fiber is placed inside a humidity-controlled glove box to prevent it from absorbing any new moisture. While inside the glove box, the fiber is inserted into a loose barrier tubing that isolates the fiber yet is still thin enough to provide adequate thermal transfer. The tubing can be further purged with various gases while it is inside the glove box to provide additional moisture isolation.
This innovation is particularly useful for fiber optic systems that measure temperature and that identify any temperature stratifications within cryogenic liquids.
Why It Is Better
This process seals sensor fibers from environmental moisture, enabling fiber optic sensing systems to operate reliably in humid environments. The innovation eliminates erroneous readings that can occur due to moisture collection on the fiber sensors.
For more information about the full portfolio of FOSS technologies, see DRC-TOPS-37 or visit https://technology-afrc.ndc.nasa.gov/featurestory/fiber-optic-sensing

Improved Ground Collision Avoidance System
This critical safety tool can be used for a wider variety of aircraft, including general aviation, helicopters, and unmanned aerial vehicles (UAVs) while also improving performance in the fighter aircraft currently using this type of system.
Demonstrations/Testing
This improved approach to ground collision avoidance has been demonstrated on both small UAVs and a Cirrus SR22 while running the technology on a mobile device. These tests were performed to the prove feasibility of the app-based implementation of this technology. The testing also characterized the flight dynamics of the avoidance maneuvers for each platform, evaluated collision avoidance protection, and analyzed nuisance potential (i.e., the tendency to issue false warnings when the pilot does not consider ground impact to be imminent).
Armstrong's Work Toward an Automated Collision Avoidance System
Controlled flight into terrain (CFIT) remains a leading cause of fatalities in aviation, resulting in roughly 100 deaths each year in the United States alone. Although warning systems have virtually eliminated CFIT for large commercial air carriers, the problem still remains for fighter aircraft, helicopters, and GAA.
Innovations developed at NASAs Armstrong Flight Research Center are laying the foundation for a collision avoidance system that would automatically take control of an aircraft that is in danger of crashing into the ground and fly it—and the people inside—to safety. The technology relies on a navigation system to position the aircraft over a digital terrain elevation data base, algorithms to determine the potential and imminence of a collision, and an autopilot to avoid the potential collision. The system is designed not only to provide nuisance-free warnings to the pilot but also to take over when a pilot is disoriented or unable to control the aircraft.
The payoff from implementing the system, designed to operate with minimal modifications on a variety of aircraft, including military jets, UAVs, and GAA, could be billions of dollars and hundreds of lives and aircraft saved. Furthermore, the technology has the potential to be applied beyond aviation and could be adapted for use in any vehicle that has to avoid a collision threat, including aerospace satellites, automobiles, scientific research vehicles, and marine charting systems.
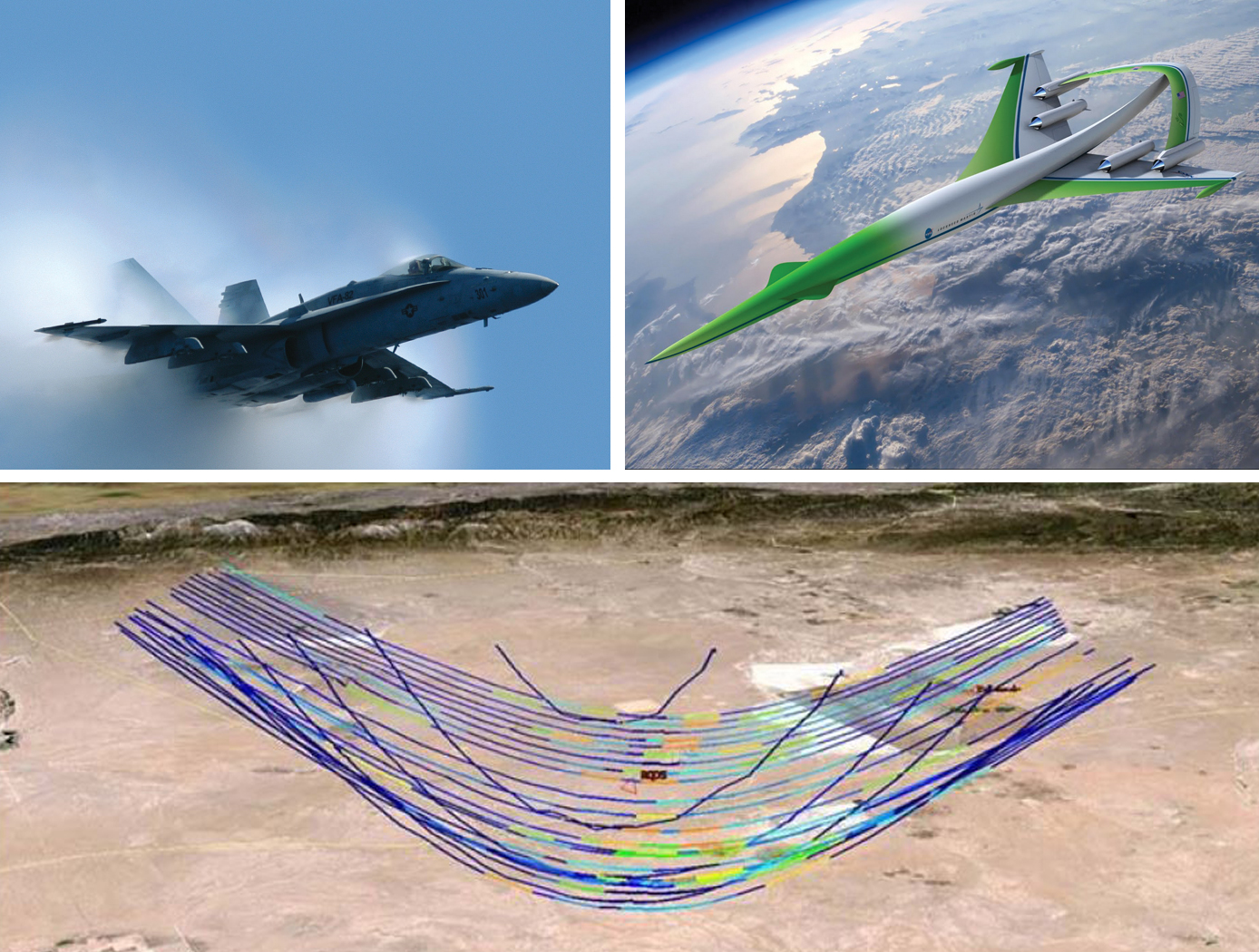
Interactive Sonic Boom Display
A supersonic shock wave forms a cone of pressurized air molecules that propagates outward in all directions and extends to the ground. Factors that influence sonic booms include aircraft weight, size, and shape, in addition to its altitude, speed, acceleration and flight path, and weather or atmospheric conditions. NASA's Real-Time Sonic Boom Display takes all these factors into account and enables pilots to control and mitigate sonic boom impacts.
How It Works
Armstrong's technology incorporates 3-dimensional (3D) Earth modeling and inputs of 3D atmospheric data. Central to the innovation is a processor that calculates significant information related to the potential for sonic booms based on an aircraft's specific operation. The processor calculates the sonic boom near a field source based on aircraft flight parameters, then ray traces the sonic boom to a ground location taking into account the near field source, environmental condition data, terrain data, and aircraft information. The processor signature ages the ray trace information to obtain a ground boom footprint and also calculates the ray trace information to obtain Mach cutoff condition altitudes and airspeeds.
Prediction data are integrated with a real-time, local-area moving-map display that is capable of displaying the aircraft's currently generated sonic boom footprint at all times. A pilot can choose from a menu of pre-programmed maneuvers such as accelerations, turns, or pushovers and the predicted sonic boom footprint for that maneuver appears on the map display. This allows pilots to select or modify a flight path or parameters to either avoid generating a sonic boom or to place the sonic boom in a specific location. The system also provides pilots with guidance on how to execute a chosen maneuver.
Why It Is Better
No other system exists to manage sonic booms in-flight. NASA's approach is unique in its ability to display in real time the location and intensity of shock waves caused by supersonic aircraft. The system allows pilots to make in-flight adjustments to control the intensity and location of sonic booms via an interactive display that can be integrated into cockpits or flight control rooms. The technology has been in use in Armstrong control rooms and simulators since 2000 and has aided several sonic boom research projects.
Aerospace companies have the technological capability to build faster aircraft for overland travel; however, the industry has not yet developed a system to support flight planning and management of sonic booms. The Real-Time Sonic Boom Display fills this need. The capabilities of this cutting-edge technology will help pave the way toward overland supersonic flight, as it is the key to ensuring that speed increases can be accomplished without disturbing population centers.
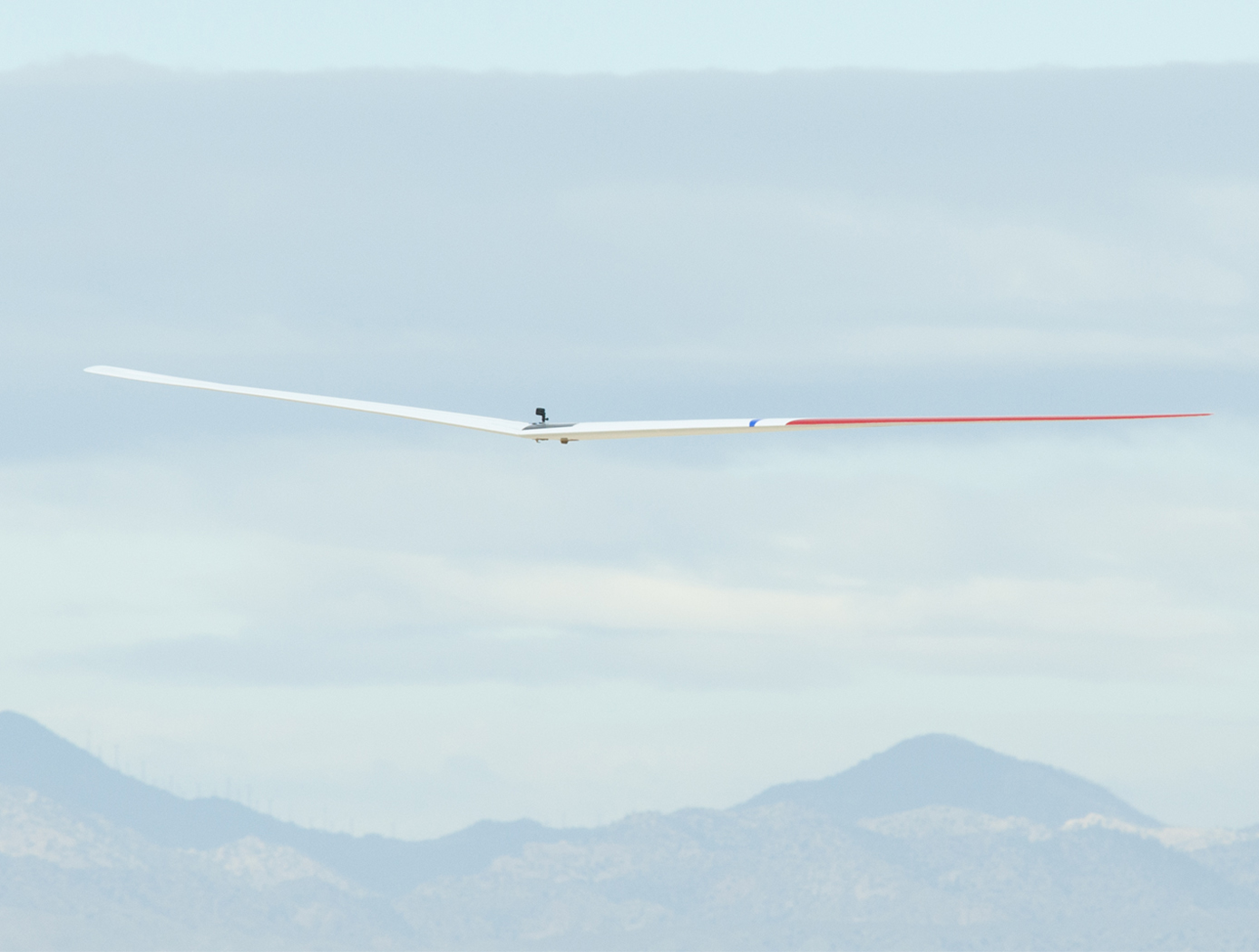
New Wing Design Exponentially Increases Total Aircraft Efficiency
Adverse yaw, present in current aircraft design, is the adverse horizontal movement around a vertical axis of an aircraft; the yaw opposes the direction of a turn. As an aircraft turns, differential drag of the left and right wings while banking contributes to aircraft yaw. Proverse yaw—yawing in the same direction as a turn—would optimize aircraft performance. Initial results from flight experiments at Armstrong demonstrated that this wing design unequivocally established proverse yaw. This wing design further reduces drag due to lift at the same time.
How It Works
The Armstrong team (supported by a large contingent of NASA Aeronautics Academy interns) built upon the 1933 research of the German engineer Ludwig Prandtl to design and validate a scale model of a non-elliptical loaded wing that reduces drag and increases efficiency.
The key to the innovation is reducing the drag of the wing through use of an alternative bell-shaped spanload, as opposed to the conventional elliptical spanload. To achieve the bell spanload, designers used a sharply tapered wing, with 12 percent less wing area than the comparable elliptical spanload wing. The new wing has 22 percent more span and 11 percent less area, resulting in an immediate 12 percent drag reduction.
Furthermore, using twist to achieve the bell spanload produces induced thrust at the wing tips, and this forward thrust increases when lift is increased at the wingtips for roll control. The result is that the aircraft rolls and yaws in the same direction as a turn, eliminating the need for a vertical tail. When combined with a blended-wing body, this approach maximizes aerodynamic performance, minimizes weight, and optimizes flight control.
Why It Is Better
Conventional aircraft make use of elliptical loaded wings to minimize drag. However, achieving aircraft stability and control in conventional elliptical wings produces a strong adverse yaw component in roll control (i.e., the aircraft will yaw the opposite direction with application of roll control). Therefore, a vertical tail or some other method of direct yaw control is required, such as split elevons for use as drag rudders. The use of elliptical wings also results in a suboptimal amount of structure to carry the integrated wing bending moment.
Adopting the bell-shaped spanload change results in an immediate 12 percent drag reduction. In addition, optimization of the overall aircraft configuration is projected to achieve additional significant overall performance increases.
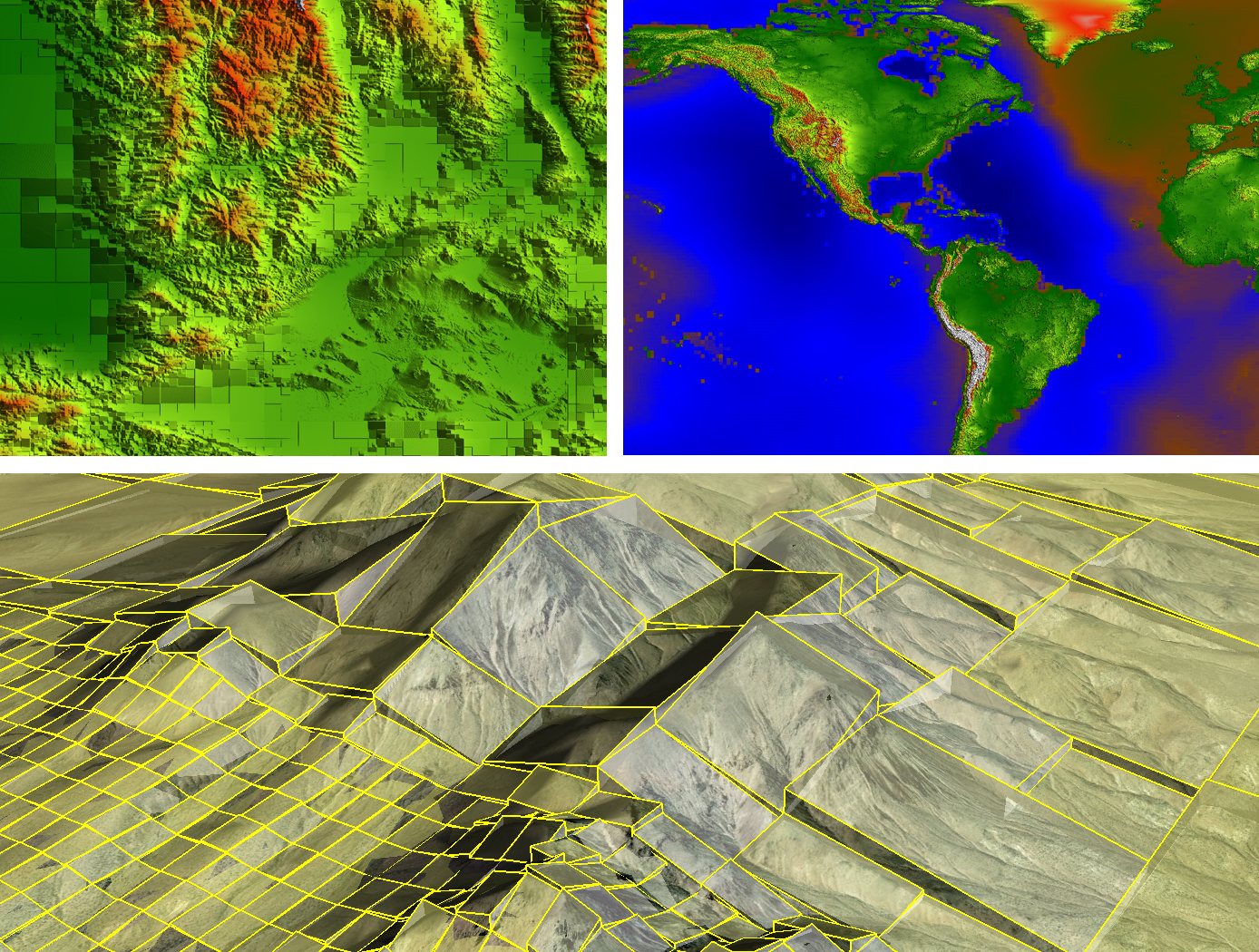
Real-Time, High-Resolution Terrain Information in Computing-Constrained Environments
NASA Armstrong collaborated with the U.S. Air Force to develop algorithms that interpret highly encoded large area terrain maps with geographically user-defined error tolerances. A key feature of the software is its ability to locally decode and render DTMs in real time for a high-performance airplane that may need automatic course correction due to unexpected and dynamic events. Armstrong researchers are integrating the algorithms into a Global Elevation Data Adaptive Compression System (GEDACS) software package, which will enable customized maps from a variety of data sources.
How It Works
The DTM software achieves its high performance encoding and decoding processes using a unique combination of regular and semi-regular geometric tiling for optimal rendering of a requested map. This tiling allows the software to retain important slope information and continuously and accurately represent the terrain. Maps and decoding logic are integrated into an aircraft's existing onboard computing environment and can operate on a mobile device, an EFB, or flight control and avionics computer systems. Users can adjust the DTM encoding routines and error tolerances to suit evolving platform and mission requirements. Maps can be tailored to flight profiles of a wide range of aircraft, including fighter jets, UAVs, and general aviation aircraft.
The DTM and GEDACS software enable the encoding of global digital terrain data into a file size small enough to fit onto a tablet or other handheld/mobile device for next-generation ground collision avoidance. With improved digital terrain data, aircraft could attain better performance. The system monitors the ground approach and an aircraft's ability to maneuver by predicting several multidirectional escape trajectories, a feature that will be particularly advantageous to general aviation aircraft.
Why It Is Better
Conventional DTM encoding techniques used aboard high-performance aircraft typically achieve relatively low encoding process ratios. Also, the computational complexity of the decoding process can be high, making them unsuitable for the real-time constrained computing environments of high-performance aircraft. Implementation costs are also often prohibitive for general aviation aircraft. This software achieves its high encoding process ratio by intelligently interpreting its maps rather than requiring absolute retention of all data. For example, the DTM software notes the perimeter and depth of a mining pit but ignores contours that are irrelevant based on the climb and turn performance of a particular aircraft and therefore does not waste valuable computational resources. Through this type of intelligent processing, the software eliminates the need to maintain absolute retention of all data and achieves a much higher encoding process ratio than conventional terrain-mapping software. The resulting exceptional encoding process allows users to store a larger library of DTMs in one place, enabling comprehensive map coverage at all times. Additionally, the ability to selectively tailor resolution enables high-fidelity sections of terrain data to be incorporated seamlessly into a map.
View more patents