Search
optics
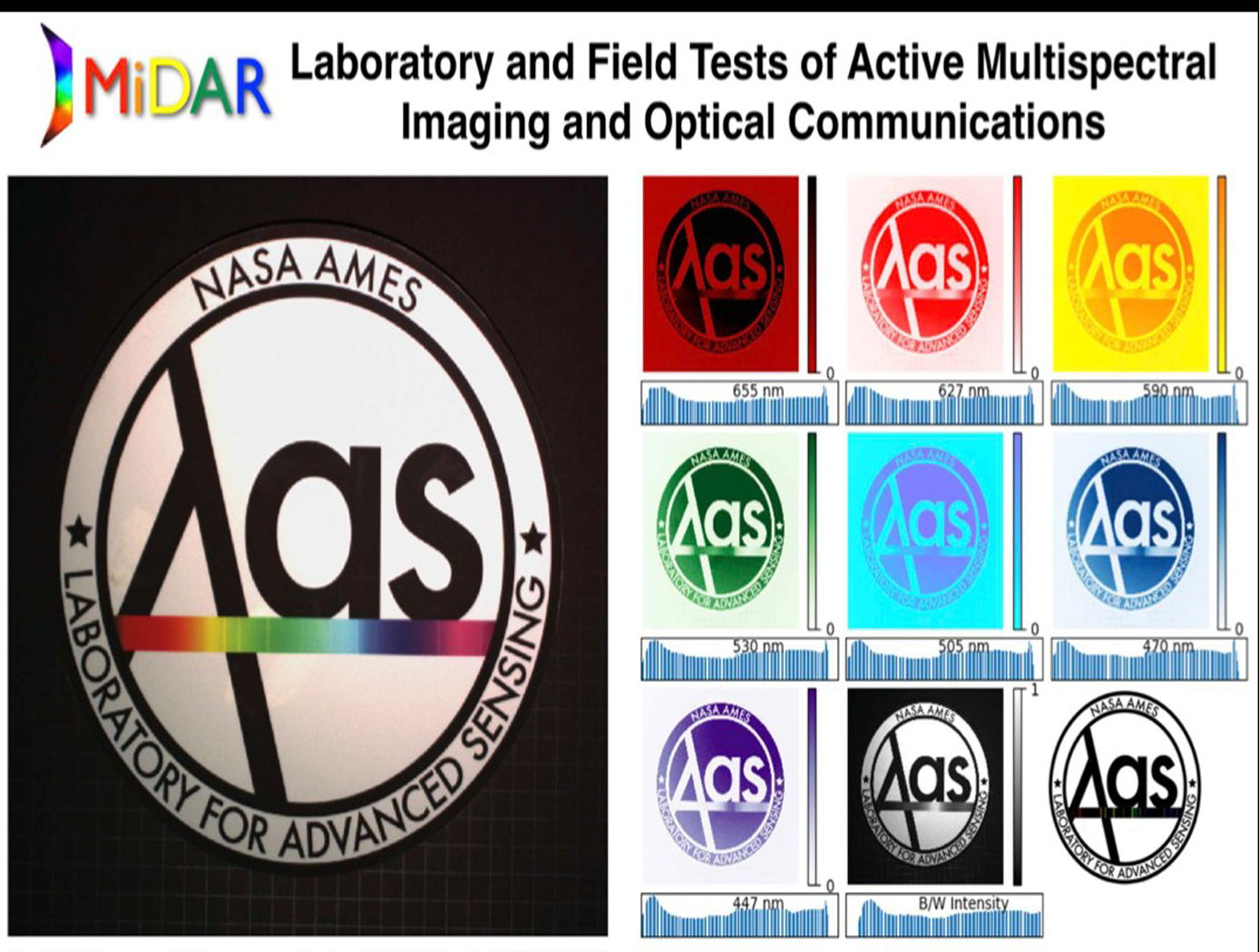
Multispectral Imaging, Detection, and Active Reflectance (MiDAR)
The MiDAR transmitter emits coded narrowband structured illumination to generate high-frame-rate multispectral video, perform real-time radiometric calibration, and provide a high-bandwidth simplex optical data-link under a range of ambient irradiance conditions, including darkness. A theoretical framework, based on unique color band signatures, is developed for multispectral video reconstruction and optical communications algorithms used on MiDAR transmitters and receivers. Experimental tests demonstrate a 7-channel MiDAR prototype consisting of an active array of multispectral high-intensity light-emitting diodes (MiDAR transmitter) coupled with a state-of-the-art, high-frame-rate NIR computational imager, the NASA FluidCam NIR, which functions as a MiDAR receiver. A 32-channel instrument is currently in development.
Preliminary results confirm efficient, radiometrically-calibrated, high signal-to-noise ratio (SNR) active multispectral imaging in 7 channels from 405-940 nm at 2048x2048 pixels and 30 Hz. These results demonstrate a cost-effective and adaptive sensing modality, with the ability to change color bands and relative intensities in real-time, in response to changing science requirements or dynamic scenes. Potential applications of MiDAR include high-resolution nocturnal and diurnal multispectral imaging from air, space and underwater environments as well as long- distance optical communication, bidirectional reflectance distribution function characterization, mineral identification, atmospheric correction, UV/fluorescent imaging, 3D reconstruction using Structure from Motion (SfM), and underwater imaging using Fluid Lensing. Multipurpose sensors, such as MiDAR, which fuse active sensing and communications capabilities, may be particularly well-suited for mass-limited robotic exploration of Earth and the solar system and represent a possible new generation of instruments for active optical remote sensing.