Smooth-Walled Feed Horn
electrical and electronics
Smooth-Walled Feed Horn (GSC-TOPS-179)
A novel feed horn design that provides the capabilities of corrugated feed horns but is easier to manufacture.
Overview
NASA Goddard Space Flight Center has developed a smooth-walled feed horn that addresses the fabrication issues inherent in constructing corrugated feed horns. Corrugated feed horns offer excellent beam symmetry, main beam efficiency, and cross-polar response over wide bandwidths. However, these feed horns can be challenging to manufacture. Our technology is a smooth-walled feed horn that approximates the properties of a corrugated feed horn over a finite bandwidth, while being easier to fabricate. The novel feed horn provides diffraction-limited ~14 degree FWHM, with low cross polarization.
The Technology
The technology is a monotonically-profiled, smooth-walled scalar feed horn optimized between 33 and 45 GHz. The phase center for this horn is near the aperture and is stable in frequency. The feed horns monotonic profile is compatible with machining by progressive plunge milling in which successively more accurate tools are used to realize the feed profile. This technique has been used for individual feeds and is potentially useful for fabricating large arrays of feed horns, such as multimode Winston concentrators, direct-machined smooth-walled conical feed horns, and for dual-mode feed horns.
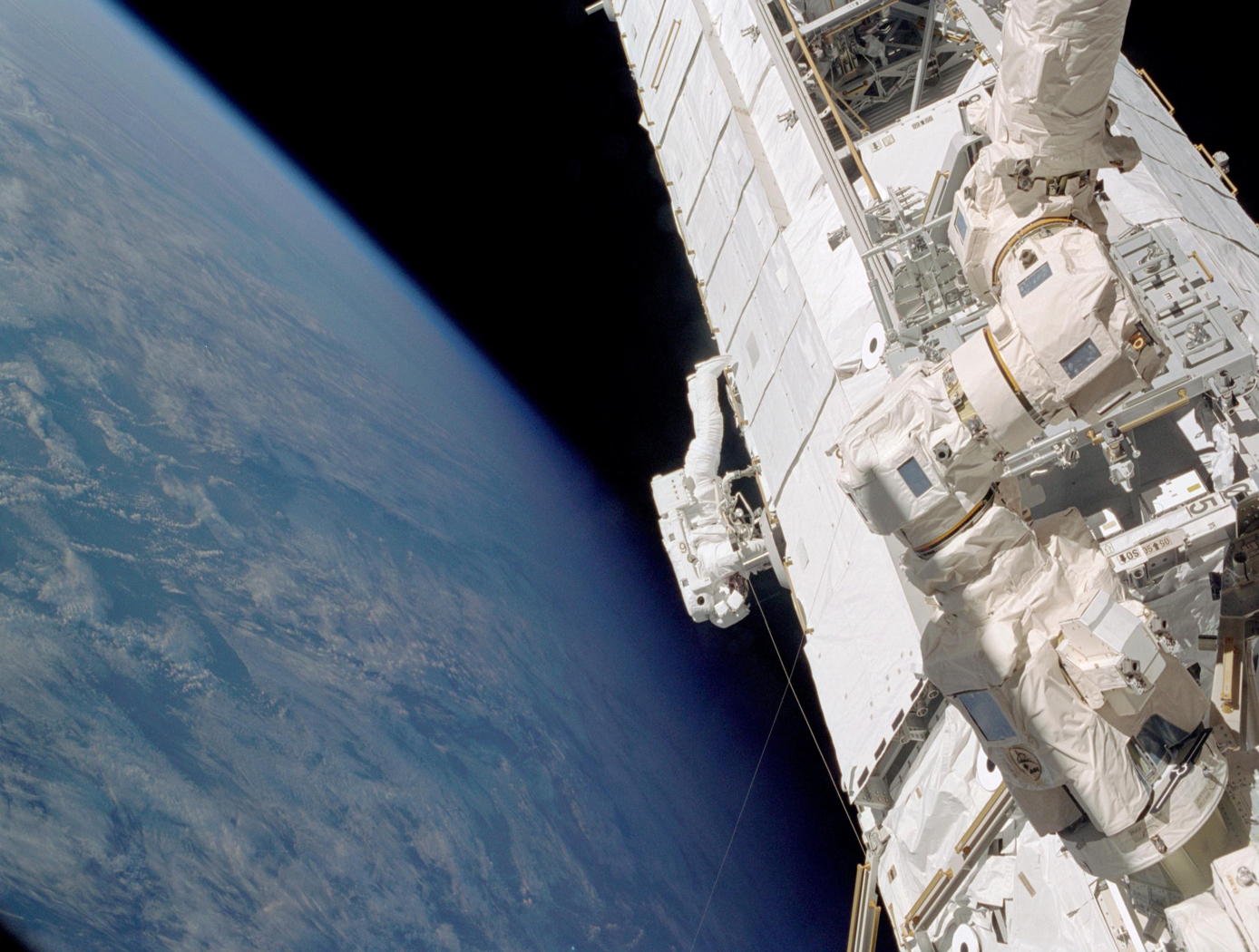
Benefits
- Easy to manufacture
- Wide bandwidth
- Low cross-polarization
Applications
- Commercial feed horn fabrication
- Radar
- Other applications involving transmission of microwaves and higher frequencies
Tags:
|
Similar Results

Quantum Cascade Laser Source and Transceiver
The QCL source addresses the challenges of inefficiency, high power consumption, and bulky designs typically associated with existing solutions. It is fabricated with 80 to 100 alternating layers of semiconductor materials, each layer only a few microns thick. These layers create a cascade effect that amplifies terahertz-energy photon generation while consuming significantly less voltage. To mitigate the natural beam dissipation of QCLs, the source is integrated with a waveguide and thin optical antenna, reducing signal loss by 50%. Additionally, the waveguide employs a flared design with a diagonal feed horn, achieving high modal confinement and increasing beam coupling efficiency to 82%, compared to 37% in conventional setups. This compact design, smaller than a U.S. quarter, fits within payload constraints and enables high-powered terahertz beams for precise spectroscopic measurements.
The terahertz transceiver enhances measurement precision by integrating two back-to-back hybrid couplers and Schottky diodes as detectors, providing a 35 dB dynamic range. Operating in the 2.0–3.2 THz frequency range, the transceiver is optimized for versatility across astrophysics, heliophysics, and planetary science applications. It seamlessly couples the QCL-generated signal onto the waveguide, ensuring stable and accurate spectroscopic data collection. This compact and energy-efficient transceiver delivers exceptional sensitivity, enabling it to analyze planetary materials, atmospheric components, and interstellar phenomena with unmatched resolution.
With its compact, tunable design and high spectral resolution, the QCL source and transceiver represents a significant advancement for remote sensing and planetary surface characterization, offering a versatile solution for both NASA and commercial applications. The QCL system is at technology readiness level (TRL) 4 (component and/or breadboard validation in lab) and is available for patent licensing.

Multi-and Wide-Band Single-Feed Patch Antenna
NASA's patch antenna technology exhibits higher operational bandwidth (on the order of 20%) than typical patch antennas (less than 10%) and can operate across integer-multiple frequency bands (e.g. S/X, C/X, S/C). Testing of the antenna design has demonstrated > 6dB of gain on both S and X bands (boresight), with an axial ratio of < 6dB and voltage standing wave ratio (VSWR) < 3:1 throughout the entire near-Earth network (NEN) operating bands (22.4GHz and 88.4GHz) with hemispherical coverage. The patch size is on the order of 10 x 10 cm and with associated electronics, is about 1 cm in height.

Lightweight, Self-Deployable Helical Antenna
NASA's newly developed antenna is lightweight (at or below 2 grams), low volume (at or below 1.2 cm3), and low stowage thickness (approx. 0.7 mm), all while delivering high performance (at or above 10 dBi gain). The antenna includes a novel design-material combination in a helical coil conformation. The design allows the antenna to compress for stowage (e.g., satellite launch), then self-deploy at the desired time in orbit.
NASA's lightweight, self-deployable helical antenna can be integrated into a thin-film solar array (or other large deployable structures). Integrating antenna elements into deployable structures such as power generation arrays allows spacecraft designers to maximize the inherently limited resources (e.g., mass, volume, surface area) available in a small spacecraft. When used as a standalone (i.e., single antenna) setup, the the invention offers moderate advantages in terms of stowage thickness, volume, and mass. However, in applications that require antenna arrays, these advantages become multiplicative, resulting in the system offering the same or higher data rate performance while possessing a significantly reduced form factor.
Prototypes of NASA's self-deployable, helical antenna have been fabricated in S-band, X-band, and Ka-band, all of which exhibited high performance. The antenna may find application in SmallSat communications (in deep space and LEO), as well as cases where low mass and stowage volume are valued and high antenna gain is required.

High Performance, All-Metal X-Band Patch Antenna
The patch antenna consists of two radiating metal patch elements, a metal feed circuit, choke rings, several alignment spacers, a SMA connector, and a mounting lid giving the antenna a total diameter of 54 mm; small enough to fit in a coffee cup. The signal is carried between the lower patch and the circuit via a coaxial transmission structure, in which the probes are the inner conductor and the antenna structure is the outer conductor. The patch antenna is constructed entirely of metal, offering rugged physical durability while delivering superior performance. This advanced material not only enables the antenna to handle higher power loads (exceeding 10 watts) but also ensures exceptional stability under demanding conditions—outperforming standard patch antennas made with traditional dielectric materials. It is also not susceptible to the manufacturing variability incurred from using dielectrics. Ideally, this metallic design also allows for reentry and reuse across missions.
The patch antenna is designed with integrated choke rings to effectively mitigate multipath signal interference, delivering an impressive front-to-back ratio of over 35 dB. Its integrated polarizer circuit enhances signal clarity and boosts overall efficiency, ensuring reliable communication in challenging environments. With support for both right- and left-handed circular polarization, the antenna achieves a co-polarization peak gain of 9 dBi and an axial ratio of less than 3 dB within a wide 50-degree orientation range. These advanced features provide superior signal performance and consistent clarity across diverse applications.
Although designed for space and planetary exploration applications, the antenna may also be valuable for terrestrial use cases with rugged conditions. The X-band patch antenna is at technology readiness level (TRL) 5 (component and/or breadboard validation in relevant environment) and is available for patent licensing.

SHEAth-based Rollable LEnticular-Shaped and Low-Stiction (SHEARLESS) Composite Booms
The SHEARLESS composite boom has a rollable structure with a large moment of inertia per unit of stored height that does not suffer from shear-derived problems. The boom is fabricated from joining two independent tape-springs front-to-front with the use of a durable seamless polymer sleeve. This sleeve allows the two parts to slide past each other during the coiling/deployment process so as to minimize shear and its derived problems. The innovation enables a lightweight structure that can be stowed on a reel without appreciable shear stresses developing in its constitutive composite parts, allowing for unprecedentedly small coiling diameters for the total thickness of the structure. As demonstrated, through specific laminate design of the two inner composite parts, the SHEARLESS composite boom can also be fabricated with a special inherent feature, bi-stability, which enables designs with minimal mechanisms and aids in deployment controllability and reliability.