Highly Thermal Conductive Polymeric Composites
materials and coatings
Highly Thermal Conductive Polymeric Composites (LAR-TOPS-175)
Carbon-based fillers for highly thermal conductive nanocomposite materials
Overview
NASA Langley Research Center has developed a method to create highly thermal conductive polymeric composites. Such materials can prove highly valuable in applications that require efficient, light-weight, and flexible thermal management solutions, such as liquid cooled ventilation garments worn by astronauts.
The Technology
There has been much interest in developing polymeric nanocomposites with ultrahigh thermal conductivities, such as with exfoliated graphite or with carbon nanotubes. These materials exhibit thermal conductivity of 3,000 W/mK measured experimentally and up to 6,600 W/mK predicted from theoretical calculations. However, when added to polymers, the expected thermal conductivity enhancement is not realized due to poor interfacial thermal transfer.
This technology is a method of forming carbon-based fillers to be incorporated into highly thermal conductive nanocomposite materials. Formation methods include treatment of an expanded graphite with an alcohol/water mixture followed by further exfoliation of the graphite to form extremely thin carbon nanosheets that are on the order of between about 2 and about 10 nanometers in thickness. The carbon nanosheets can be functionalized and incorporated as fillers in polymer nanocomposites with extremely high thermal conductivities.
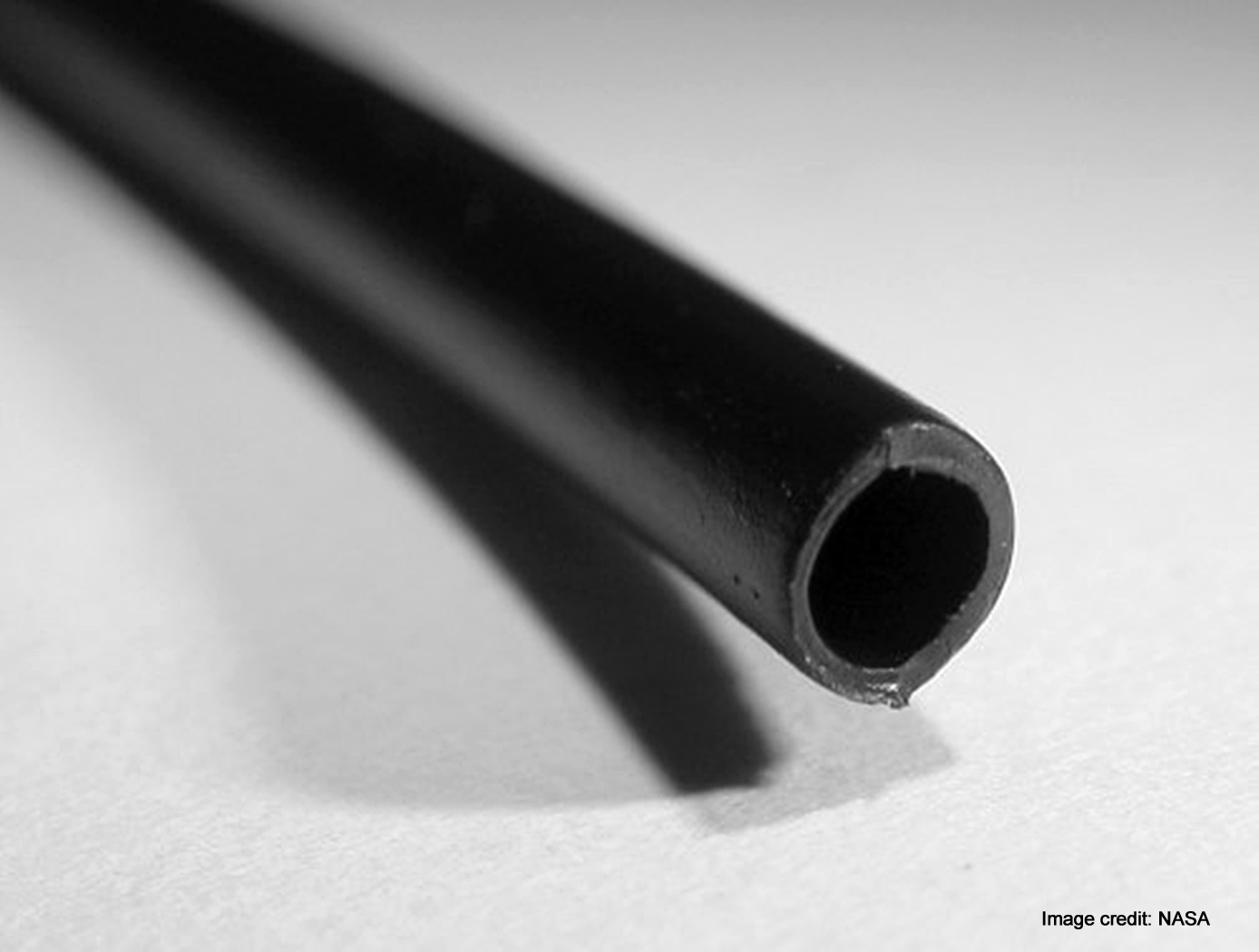
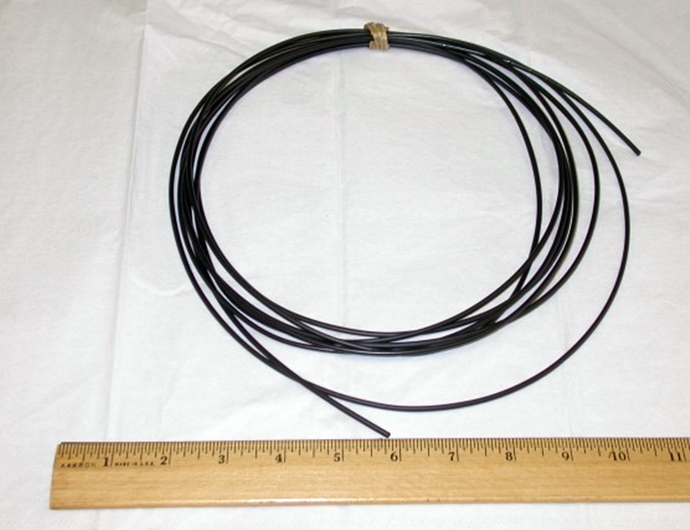
Benefits
- Relatively simple and inexpensive processing
- Can be incorporated with a wide variety of matrix materials to produce nanocomposites
Applications
- Thermally conductive clothing and components
- Liquid cooled ventilation garments
Similar Results

Advanced Materials for Electronics Insulation
Many researchers have attempted to use polymer-ceramic composites to improve the thermal and dielectric performance of polymer insulation for high voltage, high temperature electronics. However, using composite materials has been challenging due to manufacturing issues like incomplete mixing, inhomogeneous properties, and void formation. Here, NASA has developed a method of preparing and extruding polymer-ceramic composites that results in high-quality, flexible composite ribbons.
To achieve this, pellets of a thermoplastic (e.g., polyphenylsulfone or PPSU) are coated with an additive then mixed with particles of a ceramic (e.g., boron nitride or BN) as shown in the image below. After mixing the coated polymer with the ceramic particles, the blended material was processed into ribbons or films by twin-screw extrusion. The resulting ribbons are highly flexible, well-mixed, and void free, enabled by the coated additive and by using a particle mixture of micronized BN and nanoparticles of hexagonal BN (hBN).
The polymer-ceramic composite showed tunable dielectric and thermal properties depending on the exact processing method and composite makeup. Compared to the base polymer material, the composite ribbons showed comparable or improved dielectric properties and enhanced thermal conductivity, allowing the composite to be used as electrical insulation in high-power, high-temperature conditions.
The related patent is now available to license. Please note that NASA does not manufacturer products itself for commercial sale.

Aerogel Reinforced Composites
GRC's aluminosilicate aerogel composites are fabricated using a sol-gel technique. A sol is formed by hydrolyzing an alumina dispersion in acid solution; the alumina may be combined with a silicon precursor to create a sol.
Fabrics, papers, and felts are used as reinforcing fibers to form an aerogel composite. The aerogel adheres to the reinforcement without use of sizing or organic binders. (In the case of sized fabrics, the sizing is first removed by heat cleaning.) Composites can be fabricated in a batch process, impregnating individual layers of paper, felt or fabric with the precursor sol, or in a roll-to-roll process. The sol is allowed to gel, and then aged for several days prior to supercritical drying using liquid CO2. Heat treatment of the super critically dried composites can be used to tailor the alumina or Aluminosilicate crystal structure and pore size.
In contrast to commercially available insulations, GRC's innovation provides extremely low thermal conductivity (60 mW/m-K at 900°C in argon) at high temperatures, thus enabling use at higher temperatures and improving applicability. In addition, GRC's unique process provides very good adhesion of the aerogel to its reinforcing fibers in alumina papers and zirconia felts, eliminating the spalling seen in other aerogel composites. Finally, GRC's innovation demonstrates low density and extreme resilience to high temperatures and harsh conditions. Seven layers of composite material of 1.25 mm/layer produced a temperature drop of 700°C when tested in the 8-foot high-temperature wind tunnel (8 HTT) at NASA's Langley Research Center. The technology also has withstood heat tests of up to 1200°C. In combination with other insulators, it has withstood fluxes of up to 65 W/cm2, producing a temperature drop of 625°C across 8 mm.

Conductive Carbon Fiber Polymer Composite
The new composite developed by NASA incorporates PGS and CNTs to enhance its thermal conductivity while preserving the mechanical properties of the underlying carbon fiber polymer composite. NASA has also improved the composite manufacturing process to ensure better thermal conductivity not only on the surface, but also through the thickness of the material. This was achieved by adding perforations that enable the additives to spread through the composite.
The process for developing this innovative, highly thermally conductive hybrid carbon fiber polymer composite involves several steps. Firstly, a CNT-doped polymer resin is prepared to improve the matrix's thermal conductivity, which is then infused into a carbon fiber fabric. Secondly, PGS is treated to enhance its mechanical interface with the composite. Thirdly, perforation is done on the pyrolytic graphite sheet to improve the thermal conductivity through the thickness of the material by allowing CNT-doped resin to flow and better interlaminar mechanical strength. Finally, the layup of PGS and CNT-CF polymer is optimized.
Initial testing of the composite has shown significant increases in thermal conductivity compared to typical carbon fiber composites, with a more than tenfold increase. The composite also has higher thermal conductivity than aluminum alloys, with more than twice the thermal conductivity of the Aluminum 6061 typically used in the aerospace industry. For this new material, NASA has completed a proof-of-concept demonstration and work continues to use the material in a heat exchanger system and further characterize the properties including longevity and radiation impact analysis.

Layered Composite Insulation for Extreme Conditions (LCX)
The approach in developing the LCX system was to provide a combination of advantages in thermal performance, structural capability, and operations. The system is particularly suited for the complex piping, tanks, and apparatus subjected to the ambient environment common in the aerospace industry. The low-cost approach also lends the same technology to industrial applications such as building construction and chilled-water piping. The system can increase reliability and reduce life cycle costs by mitigating moisture intrusion and preventing the resulting corrosion that plagues subambient-temperature insulation systems operating in the ambient (humidity and rain) environment. Accumulated internal water is allowed to drain and release naturally over the systems normal thermal cycles. The thermal insulation system has a long life expectancy because all layer materials are hydrophobic or otherwise waterproof. LCX systems do not need to be perfectly sealed to handle rain, moisture accumulation, or condensation.
Mechanically, the LCX system not only withstands impact, vibration, and the stresses of thermal expansion and contraction, but can help support pipes and other structures, all while maintaining its thermal insulation effectiveness. Conventional insulation systems are notoriously difficult to manage around pipe supports because of the cracking and damage that can occur. Used alone or inside another structure or panel, the LCX layering approach can be tailored to provide additional acoustic or vibration damping as a dual function with the thermal insulating benefits. Because LCX systems do not require complete sealing from the weather, it costs less to install. The materials are generally removable, reusable, and recyclable, a feature not possible with other insulation systems. This feature allows removable insulation covers for valves, flanges, and other components (invaluable benefits for servicing or inspection) to be part of original designs.
Thermal performance of the LCX system has been shown to equal or exceed that of the best polyurethane foam systems, which can degrade significantly during the first two years of operation. With its inherent springiness, the system allows for simpler installation and, more importantly, better thermal insulation because of its consistency and full contact with the cold surface. Improved contact with the cold surface and better closure of gaps and seams are the keys to superior thermal performance in real systems. Eliminating the requirement for glues, sealants, mastics, expansion joints, and vapor barriers provides dramatic savings in material and labor costs of the installed system.

A New Class of Strong and Flexible Carbon Fiber Reinforced Phenolic Composites
This unique approach modifies the phenolic polymer network by adding thermoplastic molecules with flexible segments such as aliphatic carbon and siloxane. The thermoplastic molecules are terminated with bifunctional groups that can directly react with the phenolic under the curing condition to form chemical bonds. Further incorporation of these segments can be facilitated by a relay reaction of a second molecular component which can bond with both the first flexible segments and the phenolic network. The selections of flexible, thermoplastic segments are based on desired properties, which include flexibility, ablative, an charring ability, heat resistance, and low catalycity.
The modified phenolic is a truly molecular composite in which flexible segments are connected with the phenolic network through strong chemical bonds and are uniformly distributed among the networks. This feature renders a uniform toughening/strengthening effect without compromising the lightweight nature of the materials. The process is also feasible to scale up and amenable for manufacturing.