Synthesis and Development of Polyurethane Coatings Containing Fluorine Groups for Adhesive Applications
materials and coatings
Synthesis and Development of Polyurethane Coatings Containing Fluorine Groups for Adhesive Applications (LAR-TOPS-272)
Contaminant resistant coatings for extreme environments
Overview
Accumulation of insect strikes on the leading edge of airplane wings is a more serious problem than one might realize. Depending on the magnitude, such accumulation changes the aerodynamic characteristics of the wing causing a change from laminar to turbulent flow and resulting in decreased lift and increased drag. Overall, this also results in decreased fuel efficiency. According to a study published in 1950, the drag coefficient measured on an aircraft wing was determined to increase as much as 100%. Although much work has been done to mitigate this problem. Proposed solutions include elastic surfaces, coatings, soluble films, and fluid covers. Each of these approaches had significant drawbacks.
The Technology
Coatings offer an advantage over previous strategies due to ease of application, potentially negligible weight penalty, reduced environmental concerns, better economics, and continual function throughout the flight profile. In this present innovation, a particular coating has been developed that is similar to the basic component of a majority of aerospace coatings used on commercial aircraft. This coating was then sprayed from a solvent on various substrates. Once spray-coated on a substrate and dried, the coatings were then tested for adhesion mitigation of insect residues in a controlled insect impact facility propelled toward the engineered surface at approximately 150 mph. Once impacted, these coatings demonstrated hydrophobicity and a significant reduction in contaminant adhesion. The coatings were further tested in an operational environment on the eco-demonstrator Boeing 757 aircraft. The coatings resulted in lower insect accumulation than the control surface (no coating). The durability of these coatings was comparable to state-of-the-art formulations and satisfies current aircraft manufacturing requirements. These coatings likely have advantageous use in aerospace applications, wind turbine systems, and automotive industry, among other industries.
This innovation not only appears to solve a problem that has persisted, thus fulfilling an unmet need, but also comprises a new composition of matter that can lead to numerous unforeseen applications.
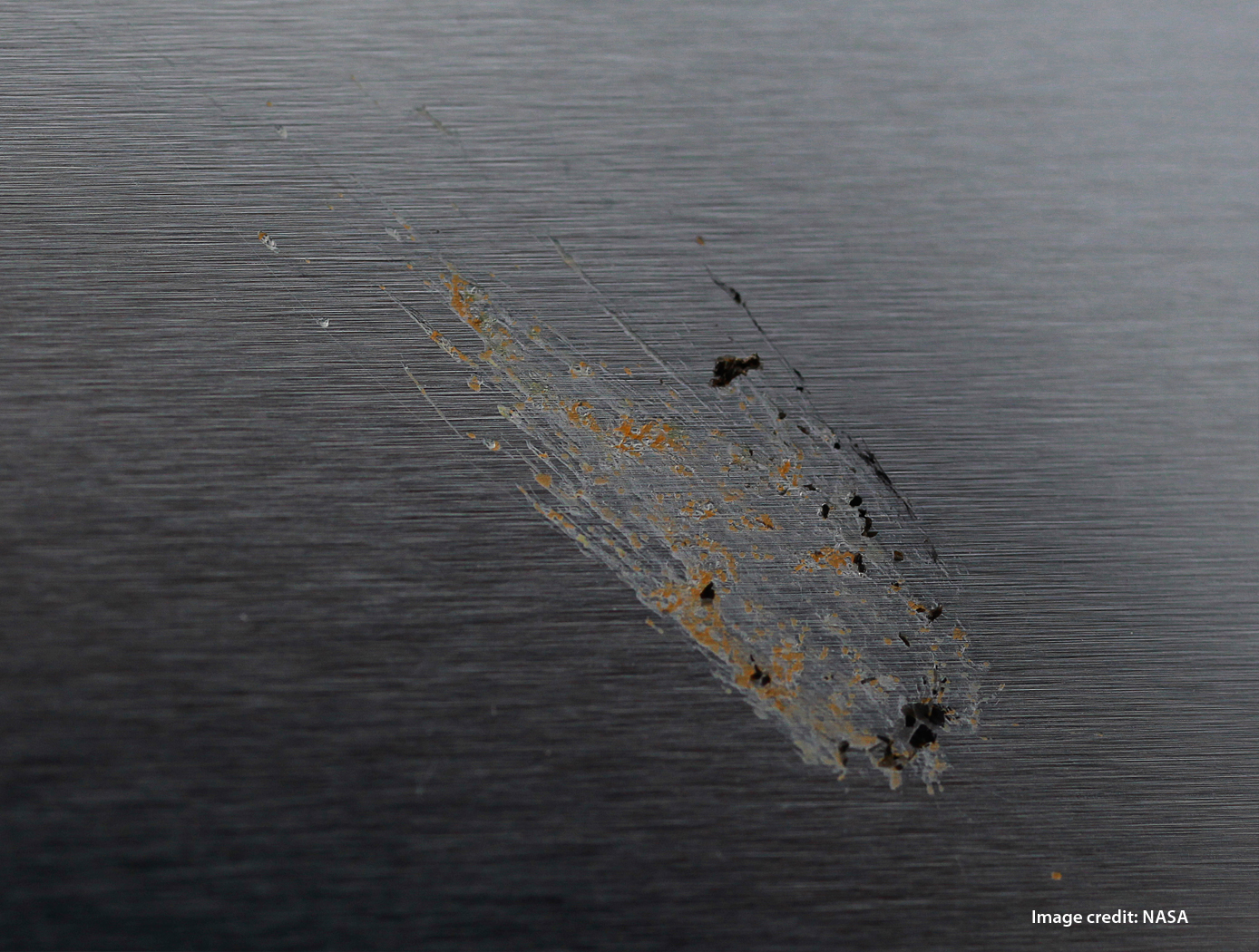
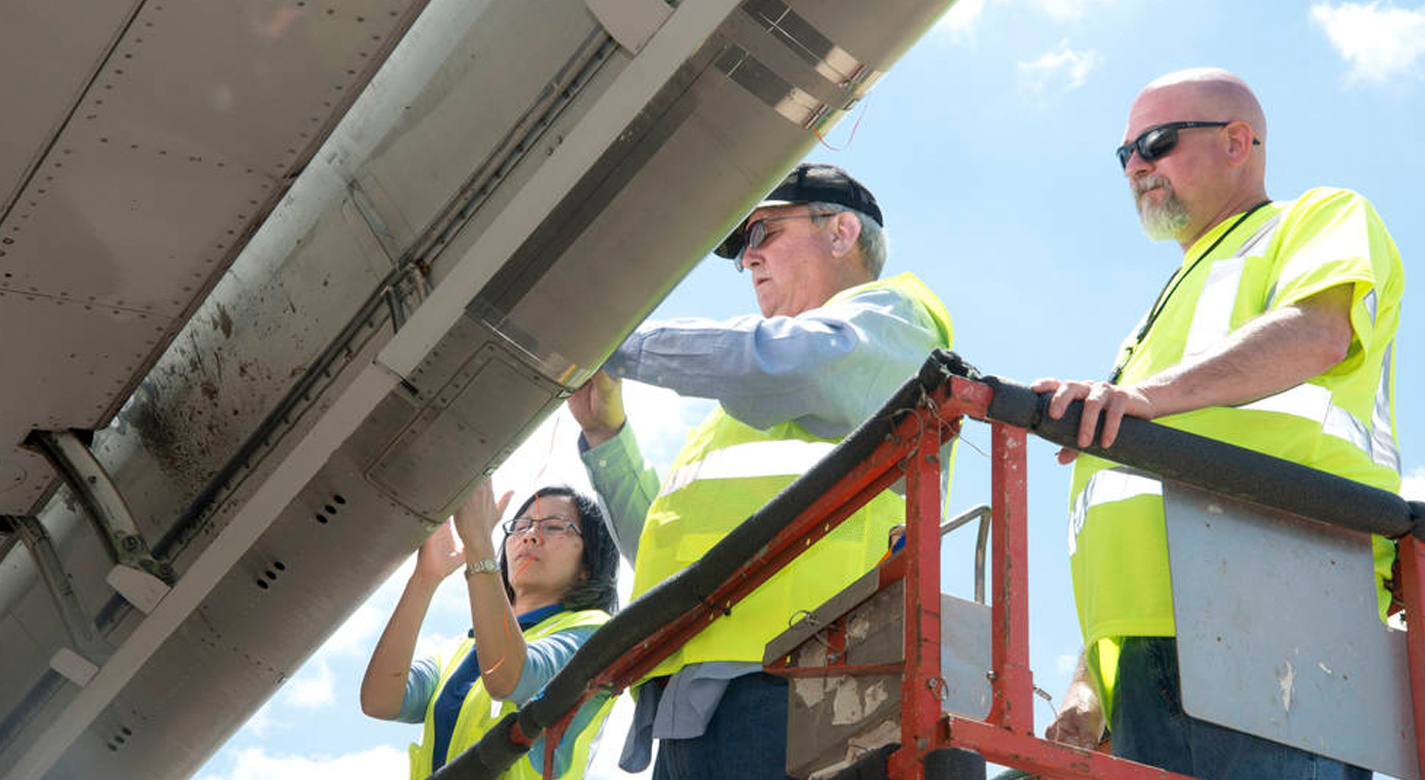
Benefits
- Elegant solution fulfills an unmet need
- Adds little weight
- Cost effective
- Saves fuel
- Improves flight safety
Applications
- Reduction of insect impact residue on aircraft
- Prevention of insect adhesion on automotive windshields
- Anti-soiling coatings
- Improved weather ability of building exteriors
- Stain & corrosion resistance
Similar Results

Hydrophobic Epoxy Coating for Insect Adhesion Mitigation
This technology is a copolymeric epoxy coating that is loaded with a fluorinated aliphatic chemical species and nano- to microscale particle fillers. The coating was developed as a hydrophobic and non-wetting coating for aerodynamic surfaces to prevent accumulation of insect strike remains that can lead to natural laminar flow disruption and aerodynamic inefficiencies. The coating achieves hydrophobicity in two ways. First, the fluorinated aliphatic chemical species are hydrophobic surface modification additives that preferentially migrate to the polymer surface that is exposed to air. Secondly, the incorporation of particle fillers produces a micro-textured surface that displays excellent resistance to wetting. Combined, these two factors increase hydrophobicity and can also be used to readily generate superhydrophobic surfaces.

Chemical and Topographical Surface Modifications for Insect Adhesion Mitigation
The technology is a method of mitigating insect residue adhesion to various surfaces upon insect impact. The process involves topographical modification of the surface using laser ablation patterning followed by chemical modification or particulate inclusion in a polymeric matrix. Laser ablation patterning is performed by a commercially available laser system and the chemical spray deposition is composed of nanometer sized silica particles with a hydrophobic solution (e.g. heptadecafluoro-1,1,2,2-tetrahydrodecyltriethoxysilane) in an aqueous ethanol solution. Both topographic and chemical modification of the substrate is necessary to achieve the desired performance.

Real-Time Drag Opti-mization Control Framework
According to the International Air Transport Association statistics, the annual fuel cost for the global airline industry is estimated to be about $140 billion in 2017. Therefore, fuel cost is a major cost driver for the airline industry. Advanced future transport aircraft will likely employ adaptive wing technologies that enable the wings of those aircraft to adaptively reconfigure themselves in optimal shapes for improved aerodynamic efficiency throughout the flight envelope. The need for adaptive wing technologies is driven by the cost of fuel consumption in commercial aviation. NASA Ames has developed a novel way to address aerodynamic inefficiencies experienced during aircraft operation. The real-time drag optimization control method uses an on-board, real-time sensor data gathered from the aircraft conditions and performance during flight (such as engine thrust or wing deflection). The sensor data are inputted into an on-board model estimation and drag optimization system which estimates the aerodynamic model and calculates the optimal settings of the flight control surfaces. As the wings deflect during flight, this technology uses an iterative approach whereby the system continuously updates the optimal solution for the flight control surfaces and iteratively optimizes the wing shape to reduce drag continuously during flight. The new control system for the flight control surfaces can be integrated into an existing flight control system. This new technology can be used on passenger aircraft, cargo aircraft, or high performance supersonic jets to optimize drag, improve aerodynamic efficiency, and increase fuel efficiency during flight. In addition, it does not require a specific aircraft math model which means it does not require customization for different aircraft designs. The system promises both economic and environmental benefits to the aviation industry as less fuel is burned.

Improved Fixed-Wing Gust Load Alleviation Device
Gust loads may have detrimental impacts on flight including increased structural and aerodynamic loads, structural deformation, and decreased flight dynamic performance. This technology has been demonstrated to improve current gust load alleviation by use of a trailing-edge, free-floating surface control with a mass balance. Immediately upon impact, the inertial response of the mass balance shifts the center of gravity in front of the hinge line to develop an opposing aerodynamic force alleviating the load felt by the wing. This passive gust alleviation control covering 33% of the span of a cantilever wing was tested in NASA Langleys low speed wind tunnel and found to reduce wing response by 30%.
While ongoing experimental work with new laser sensing technologies is predicted to similarly reduce gust load, simplicity of design of the present invention may be advantageous for certification processes. Additionally, this passive technology may provide further gust alleviation upon extending the use of the control to the entire trailing edge of the wing or upon incorporation with current active gust alleviation systems.
Importantly, the technology can be easily incorporated into to the build of nearly all fixed wing aircrafts and pilot control can be maintained through a secondary trim tab. Though challenging to retrofit, passive gust alleviation could enable use of thinner, more efficient wings in new plane design.

Green aviation - improved aerodynamic efficiency and less fuel burn
Currently, as fuel is burned, wing loading is reduced, thereby causing the wing shape to bend and twist. This wing-shape change causes the wings to be less aerodynamically efficient. This problem can be further exacerbated by modern high-aspect flexible wing design. Aircraft designers typically address the fuel efficiency goal by reducing aircraft weights, improving propulsion efficiency, and/or improving the aerodynamics of aircraft wings passively. In so doing, the potential drag penalty due to changes in the wing shapes still exists at off-design conditions. The unique or novel features of the new concepts are:
1. Variable camber flap provides the same lift capability for lower drag as compared to a conventional flap. The variable camber trailing edge flap (or leading edge slat) comprises multiple chord-wise segments (three or more) to form a cambered flap surface, and multiple span-wise segments to form a continuous trailing edge (or leading edge) curve with no gaps which could be prescribed by a mathematical function or the equivalent with boundary conditions enforced at the end points to minimize tip vortices
2. Continuous trailing edge flap (or leading edge slat) provides a continuously curved trailing edge (or leading edge) with no gaps to minimize vortices that can lead to an increase in drag.
3. The active wing-shaping control method utilizes the novel flap (or slat) concept described herein to change a wing shape to improve aerodynamic efficiency by optimizing span-wise aerodynamics.
4. An aeroelastic wing shaping method for analyzing wing deflection shape under aerodynamic loading is used in a wing-control algorithm to compute a desired command for the flap-actuation system to drive the present flap (or slat) system to the correct position for wing shaping.