Portable Compact Thermionic Power Cell
power generation and storage
Portable Compact Thermionic Power Cell (LAR-TOPS-294)
Higher power density than RTGs for long-term remote, isolated or dangerous applications
Overview
Ideal power sources for portable equipment ideally should be small, compact, lightweight, and provide continuous power with high power density. Batteries meet all but the continuous power requirement. Radioisotope thermoelectric generators (RTG) meet the continuous power condition but none of the others. However, a compact, thermionic-based cell that converts heat to electricity can meet all the above requirements . A tightly spaced array of nanometer-scale emitter points efficiently converts the heat from Plutonium-238 (238 Pu) into usable electricity. The thermionic cell uses metals with low work-function, not semiconductors, and escapes many of the RTG limitations such as lower carrier concentration and mobility, and the difficult requirement of high electrical and low thermal conductivity. It delivers an estimated 10-20% vs. 7% efficiency for an equivalent-sized, 238Pu-based RTG. Such a power cell would be ideal for remote, hazardous or otherwise isolated applications such as remote sensors/transmitters in severe environments.
The Technology
This compact thermionic cell (CTI) technology can be manufactured efficiently and economically using existing semiconductor fabrication technology. Its design consists of a top electron collector, separated by a vacuum gap from an electron emitter adjacent to the heat source, a thin plate of 238Pu enclosed by a thin-film insulator to protect the emitter and collector layers from overheating by the 238Pu. For a smart phone battery size, the invented compact thermionic (CTI) cell requires about 5 g maximum of 238Pu. Such small quantities are more readily available and producible, and could be reused for recycling when the CTI cell is dismantled. The emitter surface is topologically modified to have array of spikes, achievable using current semiconductor microfabrication technology. Various other geometries of emitter plates may also be used, such as an array of ridges. The smaller the emitter tips, the higher the voltage concentration.
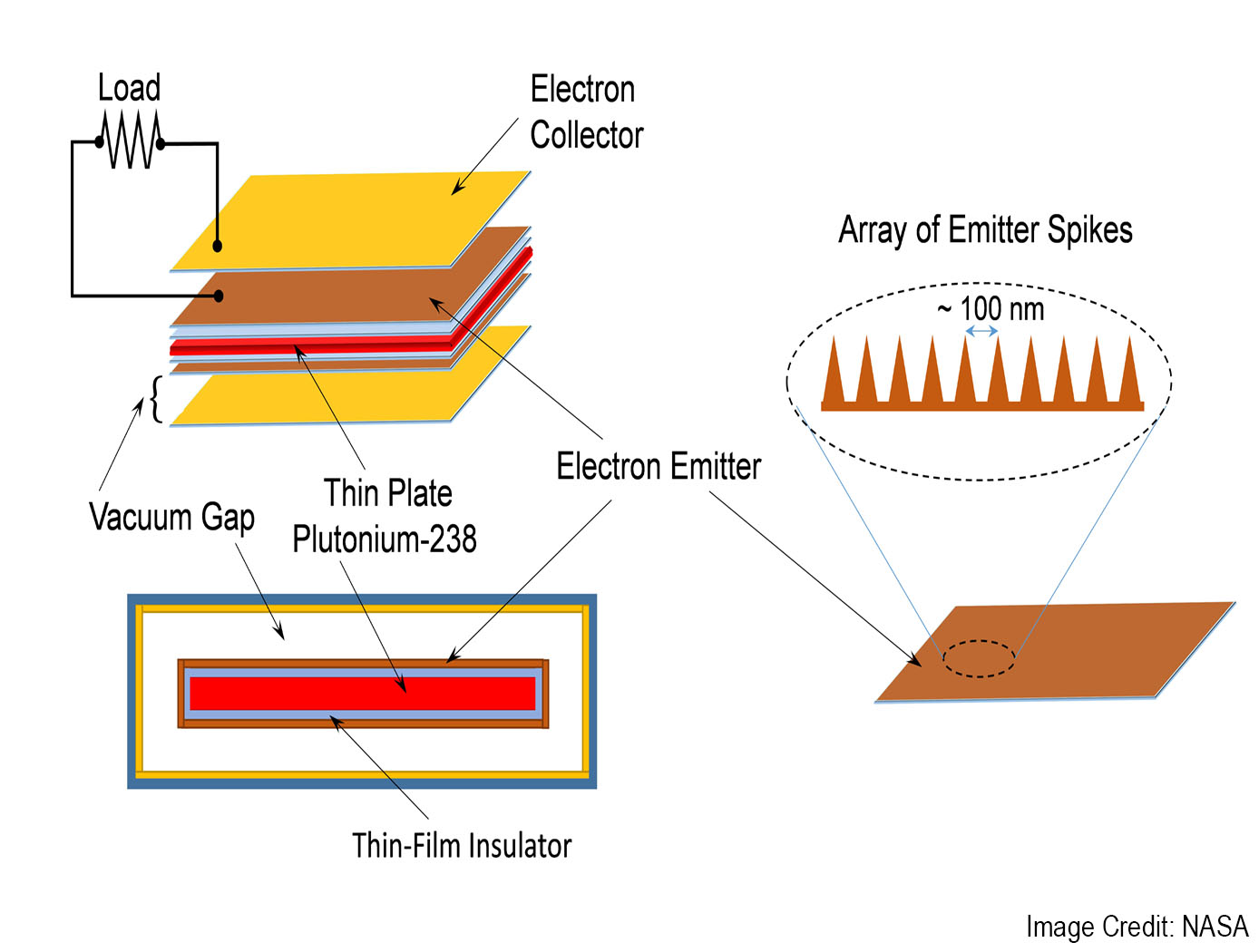
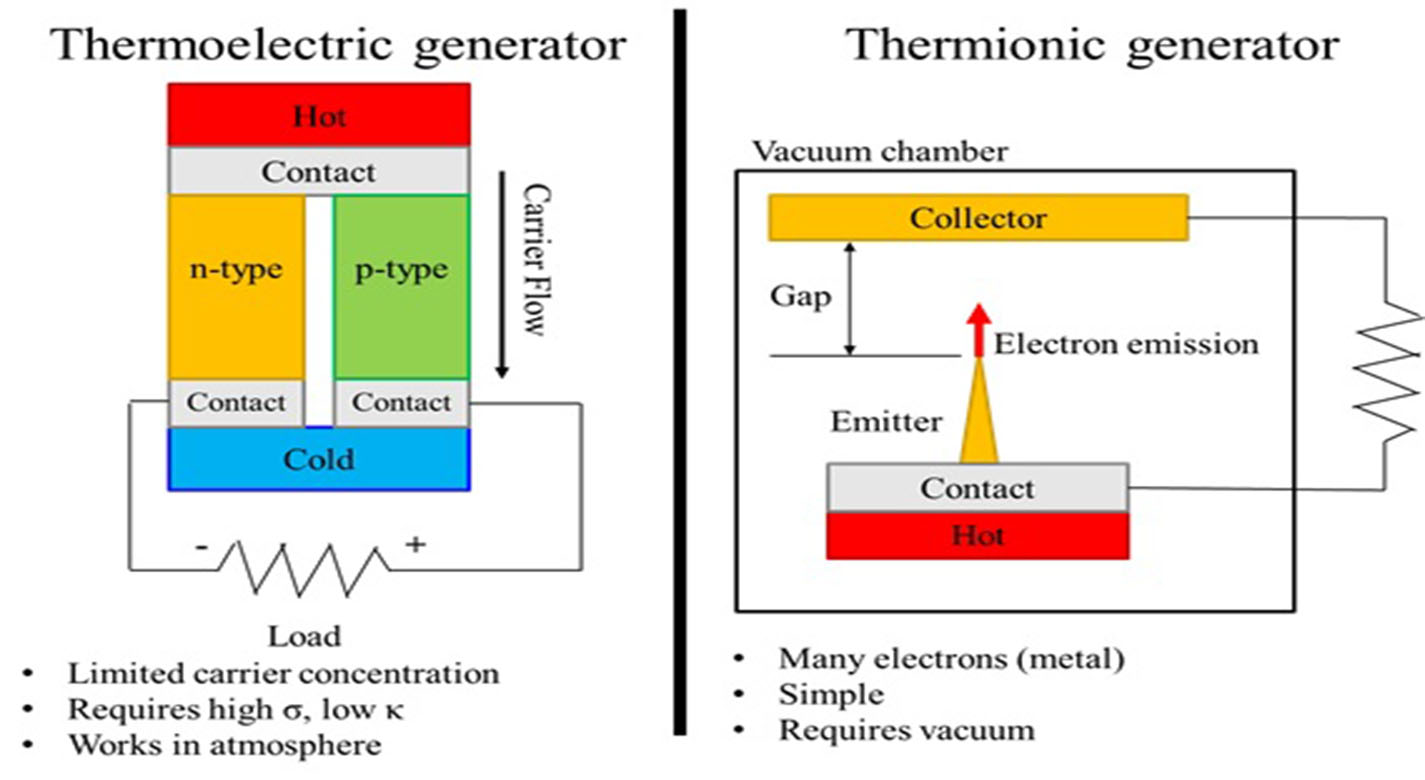
Benefits
- Thermionic emission is simpler and slightly more efficient than thermoelectric materials, with reported efficiencies of 10-20% versus 6-7% for TEG
- Adapts well-established semiconductor manufacturing techniques, lowering the initial investment costs
- Because of thin-film structures, the device can be fabricated as small as a button cell.
- Long lasting since the half-life of 238Pu decay is 87.7 years
Applications
- Any application requiring a relatively lightweight, compact, long-lived power source, including remote, hazardous (bio, chemical, radiation, environmental), dangerous, and isolated/irretrievable applications. Among them:
- Remote sensors/transmitter
- Beacons
- Small satellites
- Robots
- Drones
Technology Details
power generation and storage
LAR-TOPS-294
LAR-18860-1
LAR-18860-1-CON
Similar Results

Nuclear Thermionic and Thermoelectric Energy System
The NASA patented NTAC-TE system is a promising technology for power generation from radioactive isotopes that generate gamma rays. This will reduce the mass of unusable radioactive waste products, as NTAC-TE can use what is normally waste and harmful in order to generate electric power.
The patented integration of Cobalt-59 into fission process, which will be transmuted into Co-60, with NTAC-TE elevates the efficiency well beyond current energy harvesting methodologies considering NTAC-TE can also harness energy from Cesium-137. The gamma radiation energies from these three sources combined together are 7 MeV from the prompt fission reaction, 0.6617 MeV from the Cesium by-product of the fission process, and 1.3325 MeV from Cobalt.

Ruthenium-Doped Thermoelectric Materials
Solid state power conversion devices, such as thermoelectrics, depend upon temperature gradients for their operation. For example, aeronautic gas turbine engines maintain the necessary temperature gradients throughout their systems due to the enthalpic processes of combustion, which offers the possibility of generating electrical power for use in primary and secondary electrical systems in the aircraft. However, until now thermoelectric materials have not been able to withstand the combination of high temperatures and oxidative environments present in gas turbine engines. Glenn's innovation overcomes these limitations by using a doped oxide pyrochlore (crystal compound) semiconductor as the thermoelectric material. The material has a low thermal conductivity, which allows it to maintain a thermal gradient and sufficient electrical conductivity to produce an electromotive force. The pyrochlore allows the thermoelectric material to be present within a gas turbine engine, converting heat directly into electricity and functioning at high temperatures without oxidizing in air. Glenn's innovative thermoelectric material permits the benefits of solid-state power conversion devices to improve fuel efficiencies for a broader range of applications than has ever been possible. This innovation is in the early stages of development, and Glenn welcomes opportunities for co-development.

Silicon Oxide Coated Aluminized Polyimide Film Radiator Coating
The SiOx Coated Aluminized Polyimide Film Radiator Coating uses all the exposed surfaces on the six sides of a CubeSat as radiators. All the internal components are thermally coupled to the radiators. Waste heat from the internal components is transferred by conduction to the radiators through its aluminum structure or frame. SiOx thin film coated aluminized polyimide film is used as the radiator coating. Its total thickness is approximately 0.05 mm, which is predominately the polyimide film thickness. Polyimide film is known commercially as Kapton. The coating is bonded to the CubeSat exterior by using an acrylic transfer adhesive.
SiOx Coated Aluminized Polyimide Film Radiator Coatings absorptance and emittance can be tailored to meet the component thermal requirements by altering the SiOx thickness. Since the SiOx is a thin film, altering its thickness has no significant effect on the total thickness of the radiator coating. An indium tin oxide (ITO) thin film can be added to make the coating conductive, if needed, without affecting the absorptance or emittance. This coating, with or without ITO, can be used for various CubeSat applications. By tailoring the absorptance and emittance of this coating, external MLI blankets and active heater control are eliminated. The thermal connection between heat generating components and the battery eliminates the need for a battery heater.

Li-ion Cell Calorimeter
Li-ion batteries are an integral part of energy storage systems used in NASA's Exploration program, as well as many modern terrestrial industries. Innovators at the NASA Johnson Space Center wanted a better way to measure total and fractional heat response of specific types of Li-ion cells when driven into a thermal runaway condition. They developed a calorimeter with at least two chambers, one for the battery cell under test and at least one other chamber for receiving the thermal runaway ejecta debris. Both are designed to be structurally strong and thermally insulated. When the test cell is intentionally driven into thermal runaway, ejecta explodes into the ejecta chamber and is decelerated and collected. Thermal sensors are strategically placed throughout the chambers to collect thermal data during the test. Customized software analyzes the thermal data and determines key calorimeter parameters with a high degree of accuracy.

Internal Short Circuit Testing Device to Improve Battery Designs
Astronauts' lives depend on the safe performance and reliability of lithium-ion (Li-ion) batteries when they are working and living on the International Space Station. These batteries are used to power everything such as communications systems, laptop computers, and breathing devices. Their reliance on safe use of Li-ion batteries led to the research and development of a new device that can more precisely trigger internal short circuits, predict reactions, and establish safeguards through the design of the battery cells and packs. Commercial applications for this device exist as well, as millions of cell phones, laptops, and electronic drive vehicles use Li-ion batteries every day. In helping manufacturers understand why and how Li-ion batteries overheat, this technology improves testing and quality control processes.
The uniqueness of this device can be attributed to its simplicity. In a particular embodiment, it is comprised of a small copper and aluminum disc, a copper puck, polyethylene or polypropylene separator, and a layer of wax as thin as the diameter of one human hair. After implantation of the device in a cell, an internal short circuit is induced by exposing the cell to higher temperatures and melting the wax, which is then wicked away by the separator, cathode, and anode, leaving the remaining metal components to come into contact and induce an internal short. Sensors record the cell's reactions. Testing the battery response to the induced internal short provides a 100% reliable testing method to safely test battery containment designs for thermal runaway.
This jointly developed and patented technology is available for your company to license and develop into a commercial product. NASA does not manufacture products for commercial sale.