Beam Crossing Optical System
Optics
Beam Crossing Optical System (LAR-TOPS-346)
Novel system enabling 2-point Focused Laser Differential Interferometry
Overview
NASA's Langley Research Center has developed a Beam Crossing Optical System for use in two-point Focused Laser Differential Interferometers (FLDI) that are used in measuring density disturbances in hypersonic flows. Conventional single point FLDI systems only measure how flow fluctuates. In contrast, with the two-point system, velocity can be measured between the two points to compute spatial changes and measure fluctuating flow. In this design, laser beams propagating between the transmitter and receiver sides of the FLDI instrument remain parallel to one another so that velocity bias errors that occur in the conventional testing region with angled beams are eliminated, increasing accuracy and decreasing uncertainty of sensitivity measurements. The technology offers a lower-cost (half the cost) alternative to a Nomarski Prism approach and enables increased manipulation capabilities for the user, such that the separation distance between points and laser beam orientation can be manually adjusted with ease.
The Technology
The conventional approaches for measuring focused laser differential interferometry either use a single-point mechanism that cannot calculate velocity or a system that creates non-parallel beams in the testing zone, causing differences in time to travel between beams throughout the testing zone, adding a level of uncertainty to velocity measurements.
For this technology, the inventors determined that the best approach is to use a method that ensures all laser beams propagating between the transmitter and receiver sides of the instrument are parallel to one another. This is done by crossing two orthogonally polarized beams at a Wollaston prism located just ahead of the field lens on the transmitter side of the FLDI. The polarization orientation of the two crossing beams must be at ±45 degrees to one another so that the Wollaston prism can further split the beams by a small angle (this gives the instrument its sensitivity to density fluctuations at each measurement point).
The use of wedge prisms (that comprise the beam crossing system) to redirect the split beams such that they cross the optical axis minimizes any distortion imparted to the beams. This is in contrast to the use of a spherical focusing lens to redirect the split beams, which can impart undesirable distortions to the beams and affect the focusing properties of the FLDI instrument between its transmitter and receiver sides.
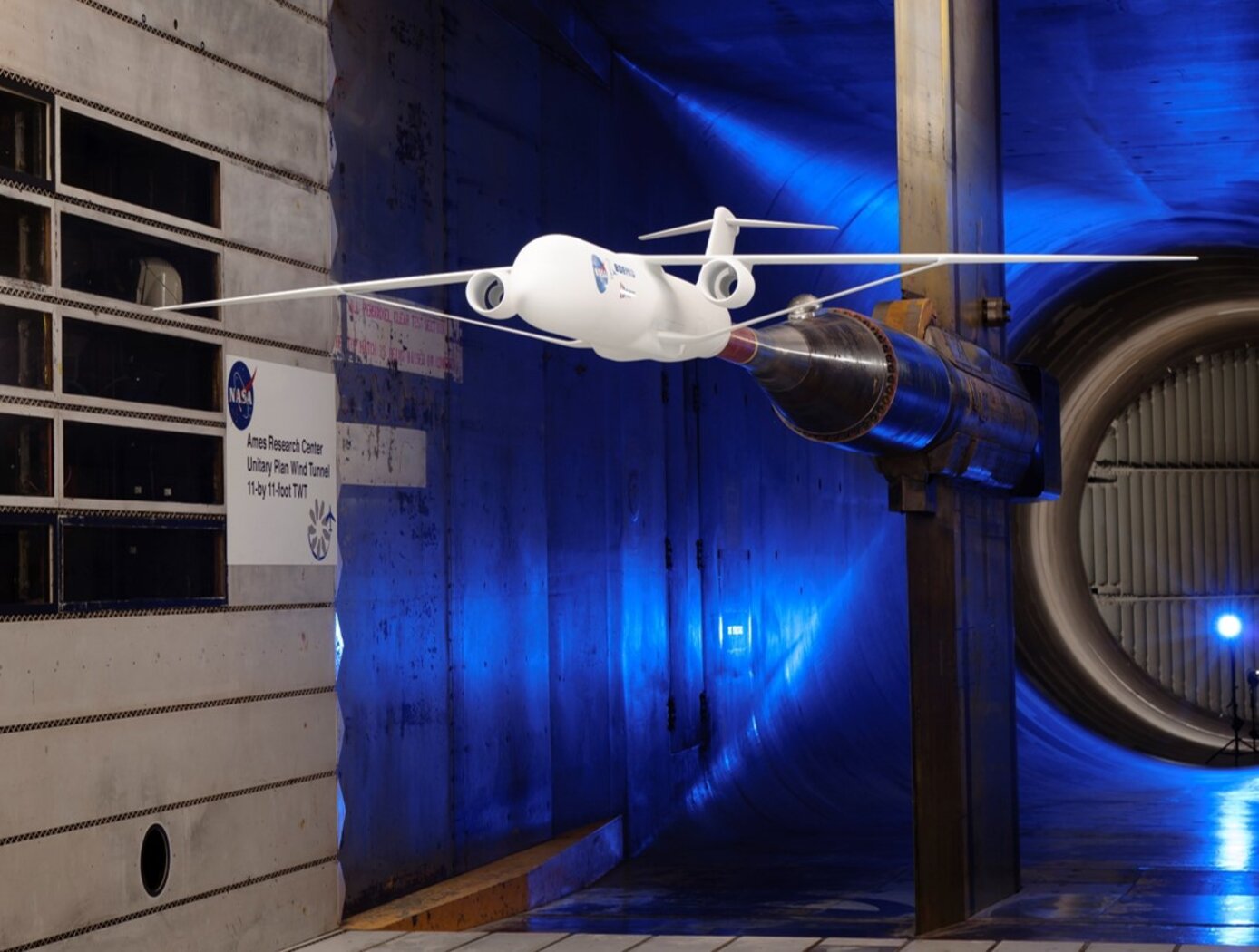
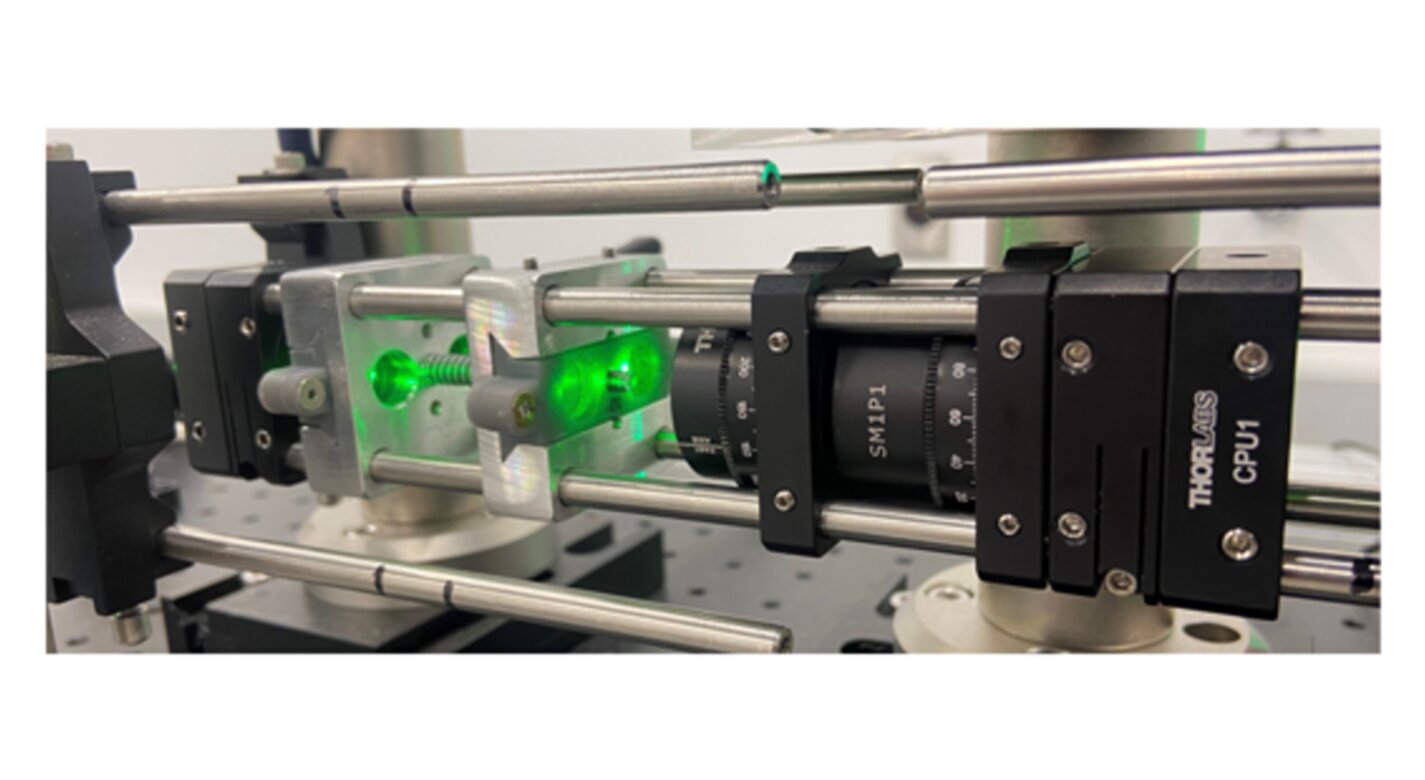
Benefits
- Enables simultaneous two-point measurement capability: Easily incorporated into a conventional single-point FLDI instrument to offer two-point measurements
- Eliminates velocity bias errors: Ensures the laser beams propagating between the transmitter and receiver sides of the FLDI instrument remain parallel to one another
- Permits tunable adjustments: The laser beams remain symmetric about the FLDI instrument’s optical axis, enabling the user to quickly adjust the orientation of the laser beams by simple rotation of the proposed optical system technology
- Provides a more cost-effective approach to FLDI: The hardware of the new NASA system costs 50% of Nomarski Prism
- Promotes user manipulation of separation distance: Design allows for easy-to-make changes to precisely align the crossing point of the beams
- Polarizing prism flexibility: The relative rotation of the wedged prism pairs allows the system to operate as a variety of adjustable polarizing prisms (e.g., Nomarski, Wollaston, Rochon, beam displacer, etc.)
Applications
- Medical Research: Differential Interference Contrast (DIC) Microscopy for cell investigation
- Gas Leak Monitoring: Use in Laser-Induced Thermal Acoustics (LITA) for gas sensing
- Aerospace and Aviation: Supersonic wind tunnel testing for various applications in aircraft development
- Academic Research: Fundamental physics research of Focused Laser Differential Interferometry (FLDI)
- Global Doppler: Two-component Doppler velocimetry with planar laser-induced-fluorescence (PLIF) for measurements in wavelength shifts
Tags:
|
Similar Results

Optical Mass Sensor for Multi-Phase Flows
Unlike commercial turbine and Venturi-type sensors, which are flow intrusive and prone to high error rates, NASA's new flow sensor technology uses an optical technique to precisely measure the physical characteristics of a liquid flowing within a pipe. It generates a reading of the flows density, which provides a highly accurate mass flow measurement when combined with flow velocity data from a second optical sensor.
NASA's sensor technology provides both a void fraction measurement, which is a measurement of the instantaneous gas/liquid percentage of a static volume and a quality measurement, which is the fraction of flow that is vapor as part of a total mass flow. It also provides a direct measurement of the gas/liquid concentration within the flow, making it suited for real-time measurement of multi-phase flows.
The technology was originally developed to accurately determine the flow rates and tank levels of multi-phase cryogenic fuels used on various NASA vehicles including the Space Shuttle and in ground-based propulsion testing. It can also be used for a wide range of gas/liquid ratios, flows with complex cross sectional profiles, flows containing bubbles or quasi-solids, and essentially any liquid, gas, or multi-phase flow that can be optically characterized. Because it is insensitive to position, the new technology also has potential for use in zero-gravity tank level sensors.

Pulsed 2-Micron Laser Transmitter
The new NASA LaRC Pulsed 2-Micron Laser Transmitter for Coherent 3-D Doppler Wind Lidar Systems is an innovative concept and architecture based on a Tm:Fiber laser end-pumped Ho:YAG laser transmitter. This transmitter meets the requirements for space-based coherent Doppler wind lidar while reducing the mission failure risks. A key advantage of this YAG based transmitter technology includes the fact that the design is based on mature and low-risk space-qualified YAG host crystal. The transmitter operates at a 2096 nm wavelength using Ho:YAG, resulting in high atmospheric transmission (>99%), versus a transmitter operating at 2053 nm using co- doped Tm:Ho:LuLiF, which suffers limited transmission (90%) due to water vapor interference. In-band pumping through Tm:Fiber pump Ho:YAG architecture offers lower quantum defect from 1908 to 2096 nm (9.1%) compared to traditionally used co-doped Tm:Ho:LuLiF of 792 to 2051 nm (61%). The transmitter has an efficient pump compared to LuLF, since YAG has 27% higher pump absorption and 52% lower reabsorption of the emitted 2-micron, resulting in higher efficiency and lower heat load. Being isotropic, YAG is amenable for spatial-hole burning mitigation which supports linear cavity architecture without compromising injection seeding quality. This attribute is important in designing a compact, stable, high seeding efficiency laser. A folded linear cavity for long pulse (>200 ns), transform limited line-width (2.2 MHz) and high beam quality (M2 = 1.04) - the most critical parameters for coherent detection - are easier to achieve using YAG compared to LuLF. Lower heat load results in high repetition rate (>300 Hz) operation, which allows higher probability of wind measurements through broken clouds, off clouds, and below clouds, thus reducing errors and increasing science data product quantity and quality.

Space Optical Communications Using Laser Beams
This invention provides a new method for optical data transmissions from satellites using laser arrays for laser beam pointing. The system is simple, static, compact, and provides accurate pointing, acquisition, and tracking (PAT). It combines a lens system and a vertical-cavity surface-emitting laser VCSEL)/Photodetector Array, both mature technologies, in a novel way for PAT. It can improve the PAT system's size, weight, and power (SWaP) in comparison to current systems. Preliminary analysis indicates that this system is applicable to transmissions between satellites in low-Earth orbit (LEO) and ground terminals. Computer simulations using this design have been made for the application of this innovation to a CubeSat in LEO. The computer simulations included modeling the laser source and diffraction effects due to wave optics. The pointing used a diffraction limited lens system and a VCSEL array. These capabilities make it possible to model laser beam propagation over long space communication distances. Laser beam pointing is very challenging for LEO, including science missions. Current architectures use dynamical systems, (i.e., moving parts, e.g., fast-steering mirrors (FSM), and/or gimbals) to turn the laser to point to the ground terminal, and some use vibration isolation platforms as well. This static system has the potential to replace the current dynamic systems and vibration isolation platforms, dependent on studies for the particular application. For these electro-optical systems, reaction times to pointing changes and vibrations are on the nanosecond time scale, much faster than those for mechanical systems. For LEO terminals, slew rates are not a concern with this new system.

Fine-pointing Optical Communication System Using Laser Arrays
A new method is described for optical data transmissions from satellites using laser arrays for fine pointing of laser beams that use body pointing. It combines a small lens system and a VCSEL/Photodetector Array in a novel way to provide a fine pointing capability for laser beams that are pointed by body pointing of a CubeSat. As Fig. 1 shows, an incoming laser beam (green or blue, with rightward arrows), transmitted from a ground terminal, enters the lens system, which directs it to an element of the pixel array (gray rectangle). Each element, or pixel, consists of a VCSEL component/photodetector pair. The photodetector detects the incoming beam, and the VCSEL component returns a modulated beam to the lens system (green or blue, with leftward arrows), which sends it to the ground terminal. As the incoming beam changes direction, e.g., from the blue to the green incoming direction, this change is detected by the adjacent photodetector, and the laser paired with that photodetector is turned on to keep the outgoing laser beam on target. The laser beams overlap so that the returning beam continues to point at the ground terminal. The VCSEL component may consist of a single VCSEL or a cluster of VCSELs. Figure 2 shows the propagation of two overlapping laser beams. The system can very accurately point finely focused diffraction-limited laser beams. Also, simultaneous optical multiple access (OMA) is possible from different transceivers within the area covered by the laser array. For this electro-optical system, reaction times to pointing changes and vibrations are on the nanosecond time scale, much faster than mechanical fine pointing systems.

Optical Head-Mounted Display System for Laser Safety Eyewear
The system combines laser goggles with an optical head-mounted display that displays a real-time video camera image of a laser beam. Users are able to visualize the laser beam while his/her eyes are protected. The system also allows for numerous additional features in the optical head mounted display such as digital zoom, overlays of additional information such as power meter data, Bluetooth wireless interface, digital overlays of beam location and others. The system is built on readily available components and can be used with existing laser eyewear. The software converts the color being observed to another color that transmits through the goggles. For example, if a red laser is being used and red-blocking glasses are worn, the software can convert red to blue, which is readily transmitted through the laser eyewear. Similarly, color video can be converted to black-and-white to transmit through the eyewear.