Pulsed 2-Micron Laser Transmitter
sensors
Pulsed 2-Micron Laser Transmitter (LAR-TOPS-334)
For Coherent 3-D Doppler Wind Lidar Systems
Overview
Innovators at the NASA Langley Research Center (LaRC) have designed a Pulsed 2-Micron Laser Transmitter for Coherent 3-D Doppler Wind Lidar Systems. The design produces a compact, efficient, long-lifetime laser transmitter as needed for use in space, while also having potential applications as an airborne or ground-based wind measurement tool. The new laser transmitter leverages two-decades of research and development expertise at NASA LaRC in the field of 2-micron lasers, particularly those for coherent Doppler wind lidar remote sensing. The invention is a conductively cooled, highly efficient, pulsed 2-Micron Tm(Thulium):Fiber pumped Ho(Holmium):YAG laser transmitter that provides optimal atmospheric transmission for wind based measurements. This innovation provides a transformational improvement in weather forecasting by minimizing severe weather losses and offering better understanding of atmospheric and atmosphere-ocean processes, helping many investigations including climate change.
The Technology
The new NASA LaRC Pulsed 2-Micron Laser Transmitter for Coherent 3-D Doppler Wind Lidar Systems is an innovative concept and architecture based on a Tm:Fiber laser end-pumped Ho:YAG laser transmitter. This transmitter meets the requirements for space-based coherent Doppler wind lidar while reducing the mission failure risks. A key advantage of this YAG based transmitter technology includes the fact that the design is based on mature and low-risk space-qualified YAG host crystal. The transmitter operates at a 2096 nm wavelength using Ho:YAG, resulting in high atmospheric transmission (>99%), versus a transmitter operating at 2053 nm using co- doped Tm:Ho:LuLiF, which suffers limited transmission (90%) due to water vapor interference. In-band pumping through Tm:Fiber pump Ho:YAG architecture offers lower quantum defect from 1908 to 2096 nm (9.1%) compared to traditionally used co-doped Tm:Ho:LuLiF of 792 to 2051 nm (61%). The transmitter has an efficient pump compared to LuLF, since YAG has 27% higher pump absorption and 52% lower reabsorption of the emitted 2-micron, resulting in higher efficiency and lower heat load. Being isotropic, YAG is amenable for spatial-hole burning mitigation which supports linear cavity architecture without compromising injection seeding quality. This attribute is important in designing a compact, stable, high seeding efficiency laser. A folded linear cavity for long pulse (>200 ns), transform limited line-width (2.2 MHz) and high beam quality (M2 = 1.04) - the most critical parameters for coherent detection - are easier to achieve using YAG compared to LuLF. Lower heat load results in high repetition rate (>300 Hz) operation, which allows higher probability of wind measurements through broken clouds, off clouds, and below clouds, thus reducing errors and increasing science data product quantity and quality.
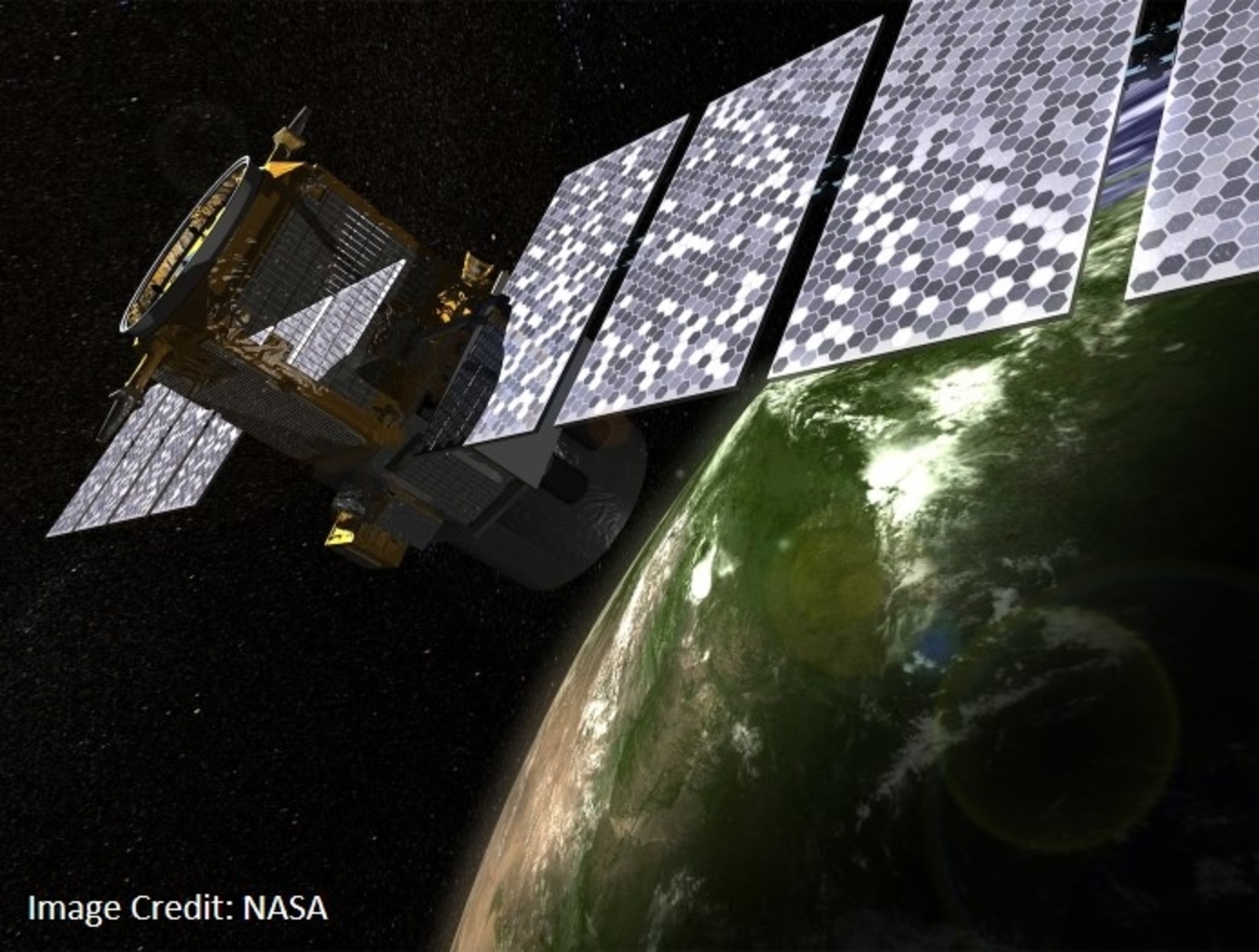
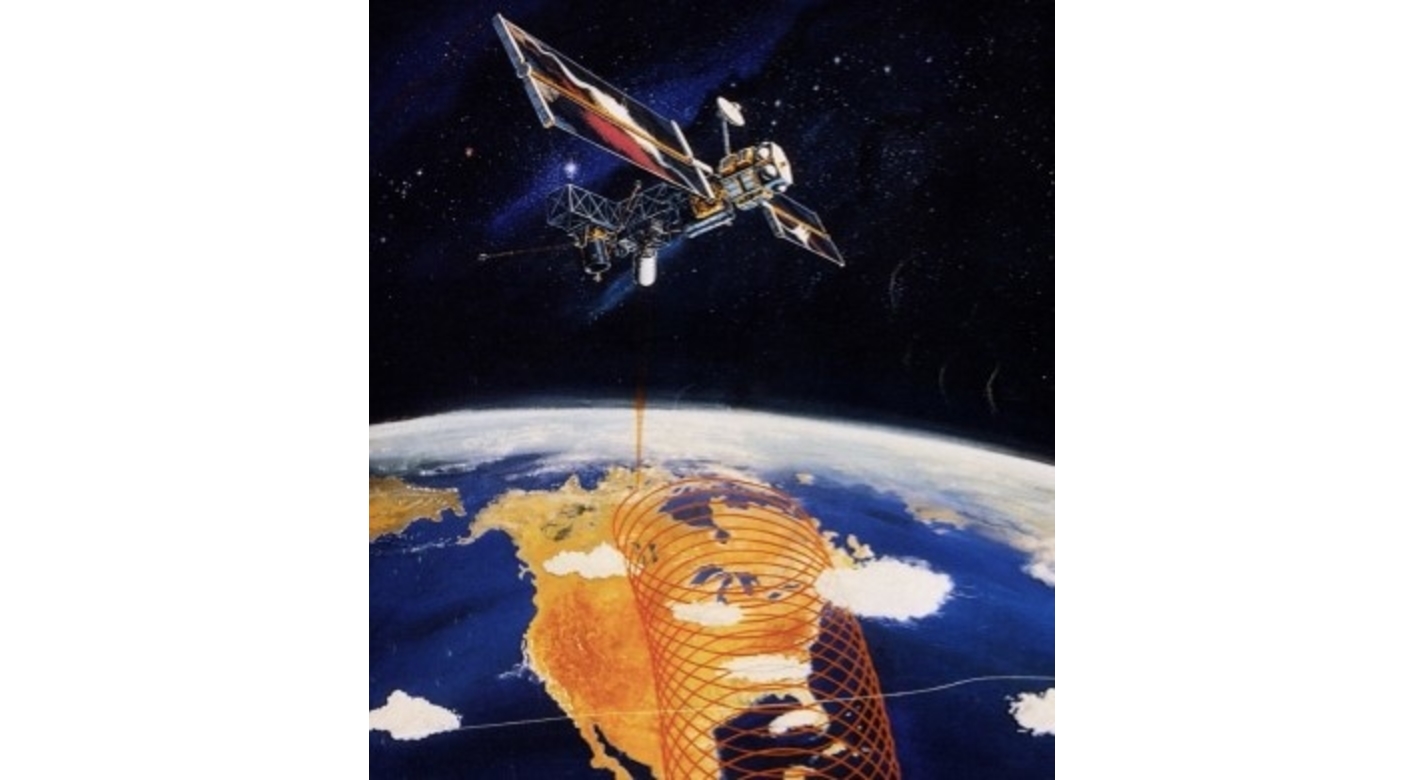
Benefits
- Enables wind speed measurements through cloud layer: this transmitter has the ability to measure wind speed under the cloud layer from space, an important missing data element in current weather models (about 60% of the Earth's surface is covered by clouds at any instant)
- Improved signal-to-noise ratio: the system achieves 200 pulses-per-second (pps), while current methods can only emit signals at 10 pps. Faster pulsing increases the likelihood that pulses can span clouds to gauge wind speed
- Compact and highly efficient: the transmitter uses a pumping frequency 10X closer to the output frequency than current methods, so much less input energy is wasted as heat. Current systems pass only 15% of the input power to output signals, while this new system passes over 95%, decreasing power and weight
Applications
- Meteorology: airborne or ground-based or space-based wind measurement for weather forecasting
- Remote sensing: coherent 3-D Doppler wind lidar from satellites
Tags:
|
Similar Results

Receiver for Long-distance, Low-backscatter LiDAR
The NASA receiver is specifically designed for use in coherent LiDAR systems that leverage high-energy (i.e., > 1mJ) fiber laser transmitters. Within the receiver, an outgoing laser pulse from the high-energy laser transmitter is precisely manipulated using robust dielectric and coated optics including mirrors, waveplates, a beamsplitter, and a beam expander. These components appropriately condition and direct the high-energy light out of the instrument to the atmosphere for measurement. Lower energy atmospheric backscatter that returns to the system is captured, manipulated, and directed using several of the previously noted high-energy compatible bulk optics. The beam splitter redirects the return signal to mirrors and a waveplate ahead of a mode-matching component that couples the signal to a fiber optic cable that is routed to a 50/50 coupler photodetector. The receiver’s hybrid optic design capitalizes on the advantages of both high-energy bulk optics and fiber optics, resulting in order-of-magnitude enhancement in performance, enhanced functionality, and increased flexibility that make it ideal for long-distance or low-backscatter LiDAR applications.
The related patent is now available to license. Please note that NASA does not manufacturer products itself for commercial sale.

Thermally-Adaptive Solid State Laser Crystal Mount
NASA’s laser mount technology introduces a unique flexible crystal mount to accommodate the dynamics of thermal expansion to eliminate unsymmetrical thermally induced mechanical stresses on the crystal. In addition, while the mount accommodates thermal expansion, it also offers fixed placement of the crystal to maintain alignment and provides continuous and uniform surface contact between the mount and crystal for rapid dissipation of heat. The mount is compatible with any heat sink reservoir.
The mount design allows unrestrained thermal expansion of the crystal in two dimensions (i.e. a- and c- axes) because of the design shown in the figure below.
The L-shape blocks also deliver cooling to the crystal by providing a path to the heat sink reservoir. The L-shape blocks are manufactured with a high thermal conductivity material such as copper. A softer material with high thermal conductivity such as indium is used to buffer the interface between the crystal and the L-shape blocks surfaces. A coolant medium acts to transfer the heat from the crystal to the cooled mount. Cooling can be provided in different ways – for example by water or by heat pipes with radiator (for use in space). The springs used to hold the laser crystal also provide the adjustment method to align the beam, and once aligned, the crystal mount is very stable.
The related patent is now available to license. Please note that NASA does not manufacture products itself for commercial sale.

Laser Linear Frequency Modulation System
For decades, frequency modulation has been used to generate chirps, the signals produced and interpreted by sonar and radar systems. Traditionally, a radio or microwave signal is transmitted toward the target and reflected back to a detector, which records the time elapsed and calculates the targets distance. Reflected signals can be heterodyned (combined) with output signals to determine the Doppler frequency shift and the target velocity. Accuracy of these systems can be enhanced by increasing the bandwidth of the chirp, but noise generated during heterodyning at high frequencies decreases the signal-to-noise ratio, increasing measurement error.
Previous attempts at laser frequency modulation that relied on adjusting the laser cavity length have resulted in only sine wave or imperfect triangle waveforms. Heterodyning of imperfect, non-linear waveforms or sine waveforms will significantly degrade the effective signal-to-noise ratio, making such systems impractical. In contrast, the current technology produces a single, high-frequency laser that is passed to an electro-optical modulator, which generates a series of harmonics. This range of frequencies is then passed through a band-pass optical filter so the desired harmonic frequency can be
isolated and directed toward the target. By modulating the electrical signal applied to the electro-optical modulator, a near perfect triangular waveform laser beam can be produced. Transmission and detection of this highly linear triangular waveform facilitates optical heterodyning for the calculation of precise frequency and phase shifts between the output and reflected signals with a high signal-to-noise ratio. By combining this information with the time elapsed, the location and velocity of the target can be determined to within 1 mm or 1 mm/s.

Wide Field Receiver Calibration Device for Micro Pulse LiDAR
Below an MPL’s minimum overlap range, the return signals are not completely in the instrument’s field of view, so the receiver only captures a portion of the backscatter laser pulse. MPL overlap ranges vary, but is usually between 4-8 km, encompassing the lower atmosphere where most aerosols reside. Commonly, correction entails recording horizontal profiles that require a ~10 km clear line-of-sight and homogenous atmospheric conditions, limiting the solution’s practicality.
In contrast, NASA’s WFR device corrects for the overlap using a second receiver co-aligned with the MPL that captures the same backscattered laser pulses as the MPL receiver, but with a ~20x wider FOV that enables a much shorter overlap range from ~6 km down to 250 m. Thus, the combination of the WFR and MPL can capture accurate signals from near surface to the stratosphere. The WFR utilizes the same detector as the MPL, enabling it to connect to the MPL data system for synced data acquisition. By eliminating the need for homogeneous horizontal measurements to determine the MPL overlap function, overlap corrections are more easily and more frequently obtained. Further, the WFR mount base was designed to easily integrate with MPLs.
NASA originally developed this device to improve accuracy of MPLs in the MPLNET, ensuring data collected are both accurate and reliable, thereby enhancing our understanding of atmospheric processes and contributing to more informed climate research and environmental modeling. The technology’s operational ease, flexibility, and cost savings are relevant to a wide range of scientific, environmental, and industrial applications. Companies that manufacture and sell MPLs may wish to offer this advanced calibration device as a product to enhance accuracy of MPL-based measurements. This NASA technology is at TRL 8 (Actual system completed and "flight qualified" through test and demonstration.) and is available for patent licensing.

Continuous Wave Laser Source for Injection Seeding
NASA's CW Laser Source for Injection Seeding uses a single laser diode (LD) to produce multiple wavelengths. Depending on the application, the seed laser may or may not be locked to a wavelength reference. For example, in atmospheric differential absorption lidar (DIAL) active remote sensing applications, the seed laser has to be locked and referenced to the species of interest using gas cells. In this context, the seed laser source is first locked to an absorption feature and the generated wavelength is used as a reference from which other offset wavelengths are generated. However, if the requirement calls only to avoid atmospheric absorption then locking may not be required.
Using this new technology, an airborne 2-micron triple pulse integrated path differential absorption (IPDA) LIDAR instrument has been developed at NASA Langley Research Center to measure the column content of atmospheric H2O and CO2 simultaneously and independently. This is achieved by transmitting three successive high-energy pulses, seeded at three different wavelengths, through the atmosphere. The three pulses are emitted 200 microseconds apart and repeated at 50 Hz. The seeding wavelengths were selected to achieve minimum measurement interference from one molecule to the other. Typically, this requires four different CW lasers for seeding. A part of that effort focused on adaptive targeting, which is based on the tuning capability of the on-line wavelength to meet a certain measurement objective depending on observational time and location. The off-line wavelength was assumed constant. The tuning capability can be achieved using the claimed seeding technique using a voltage-controlled oscillator for the on-line and fixed oscillator for the off-line.