Signal Combiner for Wideband Communication
communications
Signal Combiner for Wideband Communication (LEW-TOPS-113)
A frequency division multiplexer combined with an analog-to-digital converter increases efficiency
Overview
Innovators at NASA's Glenn Research Center have devised an efficient new method of combining primary and secondary signals with minimal loss and noise. Exploiting non-traditional uses of frequency domain multiplexers and analog-to-digital converters (ADC), Glenn's secondary signal combiner uses a frequency multiplexer in the analog domain and wideband ADC to combine electromagnetic signals (primarily MHz to GHz) with extremely low loss for both signals thereby reducing noise. With its ability to reduce system noise, this novel signal combiner delivers the best opportunity to receive a desired signal not easily distinguished from background noise. While Glenn's new technology will form part of the front-end for a new software-defined radio in ground stations, it is also poised to be an important piece in telecommunications devices, including cell phones, Wi-Fi, hot spots, satellites, and future wireless technologies.
The Technology
Through low-loss signal combination, Glenn is leading the way to optimize radio transmission remotely during self-checking routines. Glenn's signal combiner offers a simple method to minimize signal loss significantly when combining two signals. Using conventional combiners in bit-error-rate testing results in a loss of 3 to 4 dB per band, and with a directional coupler the secondary signal experiences losses of 10 dB or more. Moreover, during signal measurements, the additional components must be placed and later removed to prevent any impact to the measurement, making for a cumbersome process. Glenn's solution is to combine the primary and secondary signals in the frequency domain through the use of a frequency division diplexer/multiplexer in combination with a wideband ADC. The multiplexer selects one or more bands in the frequency domain, and the ADC performs a non-linear conversion to digital domain by folding out-of-band signals in with the primary signal. NASA makes use of subsampling a given band within the ADC bandwidth to fold it into another band of interest, effectively frequency-shifting them to a common frequency bandwidth.
Glenn's breakthrough method has two significant advantages over the conventional use of a power combiner or directional coupler in bit-error-rate testing: 1) it combines signal and noise (secondary signal) with very low loss, and 2) it enables the selection of the desired signal-to-noise ratio with no need for the later cumbersome removal of components. This streamlined process allows for invaluable in-situ or installed measurement. Glenn's novel technology has great potential for satellite, telecommunications, and wireless industries, especially with respect to equipment testing, measurement, calibration, and check-out.
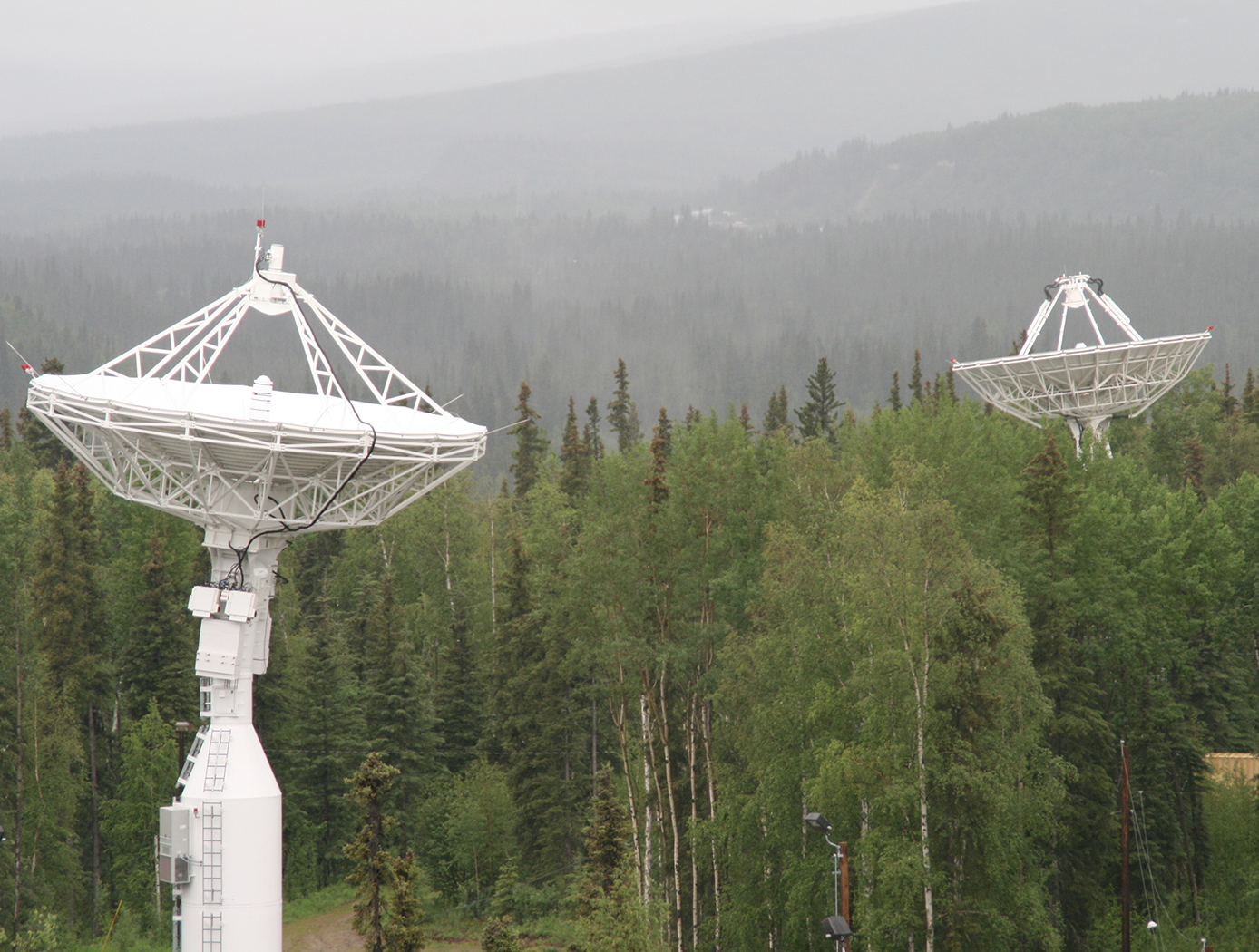
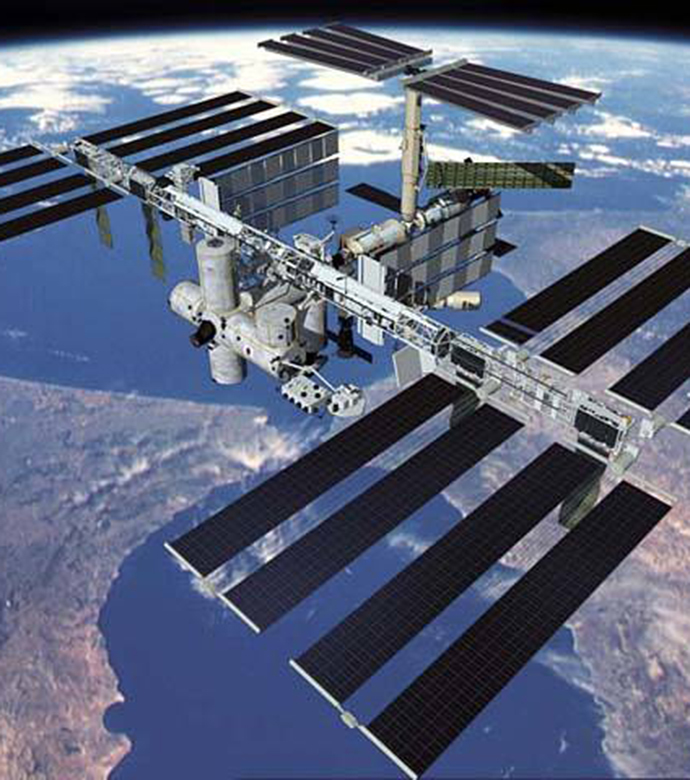
Benefits
- Efficient: Offers low distortion and improved bit-error-rate, thereby maximizing data throughput given the available spectrum
- Reduced noise: Less than 1 dB insertion loss for each frequency band
- Cost-saving: Requires very few components for effective transmission and testing
- Versatile: Can be configured to enable self-calibration, or to include a bit-rate-tester into the hardware
Applications
- Communications satellites
- Wireless communications
- Military communications
- Signal processing
- Telemetry
- Telecommunications
- Ground stations
- Software-defined radios
Similar Results

Microwave Power Combiner
MMICs are a type of integrated circuit that operates at microwave frequencies to amplify electronic signals. The system has at least two power amplifiers; input ports to receive power from the amplifiers; at least one power combiner, which receives power from each input port and combines them to produce maximized power; an output port that sends this maximized power to its destination; and an isolated port, either grounded or match-terminated, that receives no or negligible power from the combiner. The output port can be connected to a load, and can employ more than one combiner, so that the power from another combiner and an input port can be combined, for example, in a 3-way unequal power combiner.
Glenn's Ka-band demonstration power combiner has an output return loss better than 20 dB, and a high degree of isolation between the output port and the isolated port, as well as between the two input ports. When the ratio of output power for two MMICs is two-to-one, the combined efficiency is better than 90%. However, the design is not limited to a two-to-one ratio; it can be customized to any arbitrary power output ratio. This means that a low-power gallium arsenide MMIC can be combined with a high-power gallium nitride MMIC, giving designers much more flexibility. The output impedance of the MMIC power amplifier is matched directly to the waveguide impedance, without first transitioning into a transmission line. This technique eliminates the losses associated with a transition and enhances the overall efficiency. Furthermore, the MMIC power combiner is dual purpose- run in reverse it serves as a power divider. To reduce the cost and weight the combiner can be manufactured using 3-D printing and metal-plated plastic. By combining MMIC amplifiers more efficiently, Glenn's technology greatly enhances communications from near-Earth and deep space-to-Earth.

Data Transfer for Multiple Sensor Networks
High-temperature sensors have been used in silicon carbide electronic oscillator circuits. The frequency of the oscillator changes as a function of changes in the sensor's parameters, such as pressure. This change is analogous to changes in the pitch of a person's voice. The output of this oscillator, and many others may be superimposed onto a single medium. This medium may be the power lines supplying current to the sensors, a third wire dedicated to data transmission, the airwaves through radio transmission, or an optical or other medium. However, with nothing to distinguish the identities of each source, this system is useless. Using frequency dividers and linear feedback shift registers, comprised of flip flops and combinatorial logic gates connected to each oscillator, unique bit stream codes may be generated. These unique codes are used to amplitude modulate the output of the sensor (both amplitude shift keying and on-off keying are applicable). By using a dividend of the oscillator frequency to generate the code, a constant a priori number of oscillator cycles will define each bit. At the receiver, a detected frequency will have associated with it a stored code pattern. Thus, a detected frequency will have a unique modulation pattern or "voice," disassociating it from noise and from other transmitting sensors. These codes may be pseudorandom binary sequences (PRBS), ASCII characters, gold codes, etc. The detected code length and frequency are measured, offering intelligent data transfer.
This is an early-stage technology requiring additional development. Glenn welcomes co-development opportunities.

Multimode Directional Coupler
Glenn's researchers originally created the MDC to improve the beacon sources for atmospheric propagation studies. These studies are typically conducted to test atmospheric conditions to determine the signal strength needed for satellite communications. A low-power transmitter (e.g., a beacon source) is attached to the satellite, and transmits a continuous waveform (CW) signal to a receiving station on Earth. However, when a separate frequency is desired, building a new beacon source for the transmitter on the satellite - especially one that will operate at higher frequencies - presents numerous challenges. For one, a single-frequency beacon source requires a temperature-stabilized oscillator for frequency generation separate from that provided by the spacecraft receiver.
To solve such problems, Glenn's innovators fabricated the MDC from two sections of waveguide: a primary waveguide for the fundamental frequency (Ku-band), and a secondary waveguide for the harmonics (Ka-band). These sections are joined together so that precision-machined slots in the second waveguide selectively couple the harmonics, for amplification and transmission. The harmonics can then be used as an additional beacon source with very small power losses to the fundamental signal. Once the separation takes place, the second or higher harmonic can be amplified and transmitted to a station on Earth. The efficiency and performance of the MDC can be optimized through appropriate computer modeling software and currently available high-precision fabrication techniques. Without the complexity and expense involved in building separate traveling wave tube amplifiers to generate additional frequencies, Glenn's MDC enables satellites to produce multiple signals that can be received by multiple stations - a significant leap forward in satellite productivity.

High-Speed, Low-Cost Telemetry Access from Space
NASA's SDR uses Field-Programmable Gate Array (FPGA) technology to enable flexible performance on orbit. A first-generation FM-modulated transceiver is capable of operating at up to 1 Mbps downlink and 50 kbps uplink, full duplex. An FPGA performs Reed-Solomon (255,223) encoding, decoding, and bit synchronization, providing Consultative Committee for Space Data Systems (CCSDS) and Near Earth Network (NEN) telemetry protocol compatibility. The transceiver accepts data from the onboard flight computer via a source synchronous RS422 interface.
NASA's second-generation full duplex SDR, known as PULSAR (programmable ultra-lightweight system-adaptable radio, Figures 1 and 2 below) incorporates command receiver and telemetry transmitters, as well as updated processing and power capabilities. An S-band command receiver offers a max uplink data rate of 300 Kbps and built-in QPSK demodulation. X- and S-Band telemetry transmitters offer a max downlink data rate of 150 Mbps and flexible forward-error correction (FEC) using Reed-Solomon encoding (LDPC rate 7/8 and 1/2 convolution in development), and it uses QPSK modulation. The use of FEC adds an order of magnitude increase in telemetry throughput due to an improved coding gain. An onboard FPGA uses high-speed logic for uplink/downlink and encoding/decoding processes. Balloon flight testing has been conducted and is ongoing for PULSAR.

Tunable Multi-Tone, Multi-Band, High-Frequency Synthesizer
Glenn's revolutionary new multi-tone, high-frequency synthesizer can enable a major upgrade in the design of high data rate, wide-band satellite communications links, in addition to the study of atmospheric effects. Conventional single-frequency beacon transmitters have a major limitation: they must assume that atmospheric attenuation and group delay effects are constant at all frequencies across the band of interest. Glenn's synthesizer overcomes this limitation by enabling measurements to be made at multiple frequencies across the entire multi-GHz wide frequency, providing much more accurate and actionable readings.
This novel synthesizer consists of a solid-state frequency comb or harmonic generator that uses step-recovery semiconductor diodes to generate a broad range of evenly spaced harmonic frequencies, which are coherent and tunable over a wide frequency range. These harmonics are then filtered by a tunable bandpass filter and amplified to the necessary power level by a tunable millimeter-wave power amplifier. Next, the amplified signals are transmitted as beacon signals from a satellite to a ground receiving station. By measuring the relative signal strength and phase at ground sites the atmospheric induced effects can be determined, enabling scientists to gather essential climate data on hurricanes and climate change. In addition, the synthesizer can serve as a wideband source in place of a satellite transponder, making it easier to downlink high volumes of collected data to the scientific community. Glenn's synthesizer enables a beacon transmitter that, from the economical CubeSat platform, offers simultaneous, fast, and more accurate wideband transmission from space through the Earth's atmosphere than has ever been possible before.