Cascaded Offset Optical Modulator
communications
Cascaded Offset Optical Modulator (LEW-TOPS-148)
For Optical Communications
Overview
Innovators at the NASA Glenn Research Center have developed the Cascaded Offset Optical Modulator, an electro-optic subsystem used to interface a binary output Software Defined Radio (SDR) to an optical transmission system. Traditionally, this task is accomplished through the use of a Mach Zehnder Modulator (MZM), a primarily analog component. In order to generate a high-fidelity optical signal, the input electrical signal must be of equally high fidelity, which is a difficult task due to the competing requirements of the digital components and the analog modulator. The digital components required to generate the waveform contain non-idealities which degrade the extinction ratio (ER) of the optical signal. The Cascaded Offset Optical Modulator corrects these non-idealities in the electro-optic subsystem during modulation by relieving the SDR of the extreme fidelity requirements imposed by the optical modulator. This approach is valid in any optical transmission system that requires high fidelity binary pulses without a complex component.
The Technology
A unique challenge in the development of a deep space optical SDR transmitter is the optimization of the ER. For a Mars to Earth optical link, an ER of greater than 33 dB may be necessary. A high ER, however, can be difficult to achieve at the low Pulse Position Modulation (PPM) orders and narrow slot widths required for high data rates. The Cascaded Offset Optical Modulator architecture addresses this difficulty by reducing the width of the PPM pulse within the optical modulation subsystem, which relieves the SDR of the high signal quality requirements imposed by the use of an MZM. With the addition of a second MZM and a variable time delay, all of the non-idealities in the electrical signal can be compensated by slightly offsetting the modulation of the laser. The pulse output is only at maximum intensity during the overlap of the two MZMs. The width of the output pulse is effectively reduced by the offset between MZMs. Measurement and analysis of the system displayed, for a 1 nanosecond pulse width, extinction ratios of of 32.5 dB, 39.1 dB, 41.6 dB, 43.3 dB, 45.8 dB, and 48.2 dB for PPM orders of 4, 16, 32, 64, 128, and 256, respectively. This approach is not limited to deep space optical communications, but can be applied to any optical transmission system that requires high fidelity binary pulses without a complex component. The system could be used as a drop-in upgrade to many existing optical transmitters, not only in free space, but also in fiber. The system could also be implemented in different ways. With an increase in ER, the engineer has the choice of using the excess ER for channel capacity, or simplifying other parts of the system. The extra ER could be traded for reduced laser power, elimination of optical amplifiers, or decreased system complexity and efficiency.
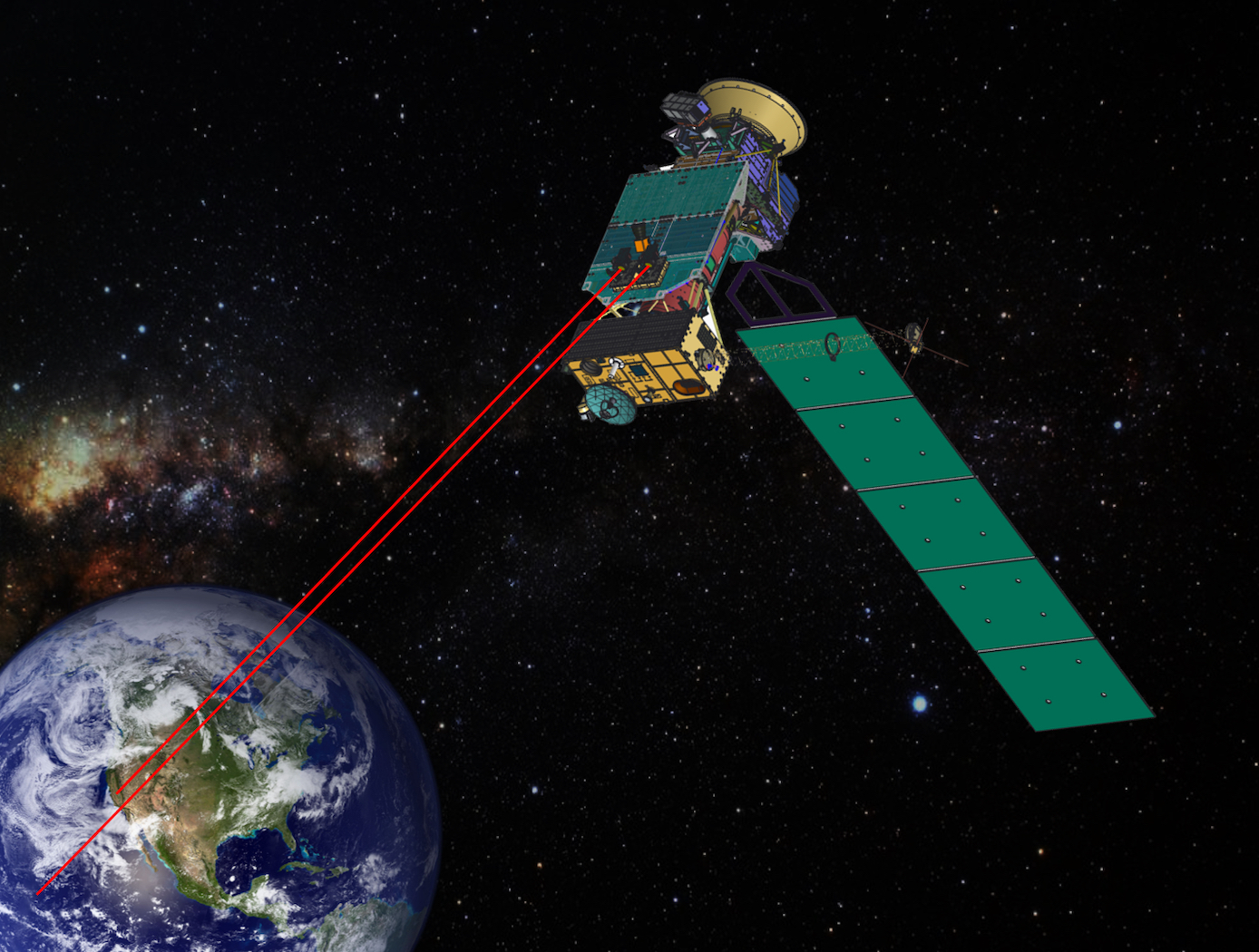
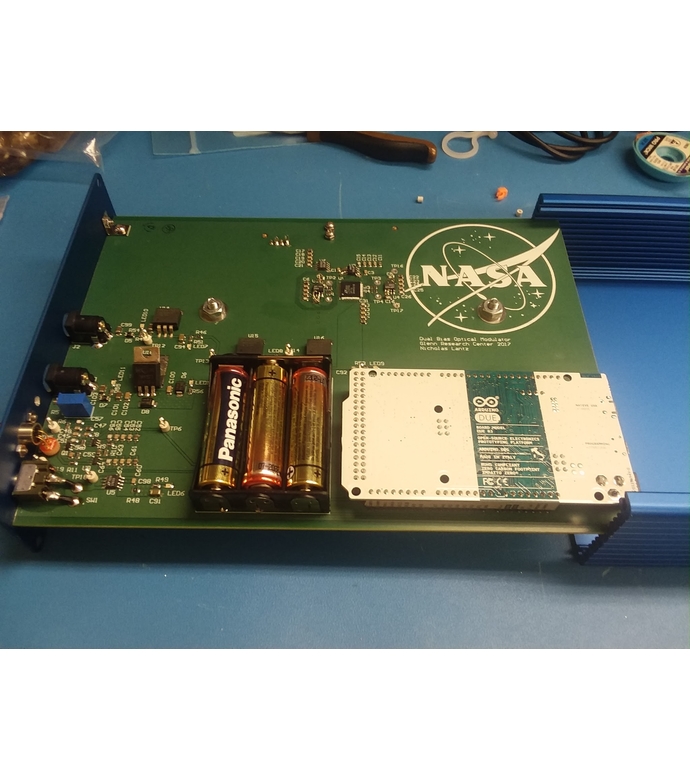
Benefits
- Reduced complexity for optical modulators in Pulse Position Modulation (PPM) systems: The Cascaded Offset Optical Modulator reduces the signal quality requirement for the SDR, resulting in a less complex and more cost effective implementation
- Increased channel capacity and reduced SWaP: This innovation simplifies the electrical portion of an electro-optic system, which can significantly increase channel capacity and reduce size, weight, and power (SWaP)
- High-quality PPM signal: The Cascaded Offset Optical Modulator has achieved a signal with a 40 dB extinction ratio for PPM32 and a 48 db extinction ration for PPM256
- Compatible with current communications infrastructure: Drop-in upgrade to many existing optical transmitters in free space and fiber
Applications
- Free-space optical communications
- Fiber-optic communications
Technology Details
communications
LEW-TOPS-148
LEW-19698-1
"Optical Software Defined Radio Transmitter Extinction Ratio Enhancement with Differential Pulse Carving," Lantz, Nicholas C., et al., February 2, 2019,
https://ntrs.nasa.gov/search.jsp?R=20190025712
https://ntrs.nasa.gov/search.jsp?R=20190025712
Tags:
|
Similar Results

Fast Optical Shutter, Chopper, Modulator, and Deflector
A laser or a light source is incident on a detector. It usually passes through a shutter that can open and then close to limit the amount of light hitting the detector. There are limitations on the speed, size and cost of such apertures. The design of this technology uses DLP mirror technology. It can rapidly deflect the incoming light beam onto an aperture, which blocks the beam path, or through the aperture, which allows it to go onto the detector. The DLP mirror in this shutter uses an aperture design that is nearly 3 orders of magnitudes faster (shorter exposure time) than similar-sized aperture using conventional commercial-off-the-shelf mechanical shutters and 1-2 orders of magnitude smaller and cheaper than higher-performing custom-made shutters that are used by a few labs around the world.
The DLP mirror is actuated via a computer controlled oscillator circuit. A laser beam directed to the mirror is either passed to a target detector, or diverted, based on the inputs from the circuit. In this manner, the DLP mirror / circuit can act as a fast shutter, modulator, or chopper for the light beam. One novel feature of this invention is the application of the DLP to divert a beam onto or off a detector for instrumentation systems.

Gated Chopper Integrator (GCI)
The gated chopper integrators function is to amplify low level signals without introducing excessive offset and noise and to do this with accurate and variable gain. The unique feature of the technology is the inherent demodulation present in the integrator which eliminates the need for filtering and allows the user to accurately vary the gain in finely graduated steps. The reduction of the offset of the amplifier is very efficient and lends itself to radiation hardened by design implementations. Since total dose can change the offset due to varying threshold voltages of CMOS transistors, the circuit adapts and compensates for any variations. The autozero integrator also adapts to its own varying offsets. The net outcome is variable, accurate gain that is very robust to supply variations, radiation effects and aging.
The technology was developed as a multi-channel thermopile signal processor. Lab measurements indicate very accurate amplification with low offset and noise.

Multimode Directional Coupler
Glenn's researchers originally created the MDC to improve the beacon sources for atmospheric propagation studies. These studies are typically conducted to test atmospheric conditions to determine the signal strength needed for satellite communications. A low-power transmitter (e.g., a beacon source) is attached to the satellite, and transmits a continuous waveform (CW) signal to a receiving station on Earth. However, when a separate frequency is desired, building a new beacon source for the transmitter on the satellite - especially one that will operate at higher frequencies - presents numerous challenges. For one, a single-frequency beacon source requires a temperature-stabilized oscillator for frequency generation separate from that provided by the spacecraft receiver.
To solve such problems, Glenn's innovators fabricated the MDC from two sections of waveguide: a primary waveguide for the fundamental frequency (Ku-band), and a secondary waveguide for the harmonics (Ka-band). These sections are joined together so that precision-machined slots in the second waveguide selectively couple the harmonics, for amplification and transmission. The harmonics can then be used as an additional beacon source with very small power losses to the fundamental signal. Once the separation takes place, the second or higher harmonic can be amplified and transmitted to a station on Earth. The efficiency and performance of the MDC can be optimized through appropriate computer modeling software and currently available high-precision fabrication techniques. Without the complexity and expense involved in building separate traveling wave tube amplifiers to generate additional frequencies, Glenn's MDC enables satellites to produce multiple signals that can be received by multiple stations - a significant leap forward in satellite productivity.

Serial Arrayed Waveguide Grating
Serial Arrayed Waveguide Grating enables higher resolution wavelength separation. Traditional AWGs split the optical signal into multiple parallel paths each with a different path length. This new approach creates the different path lengths by splitting the signal into essentially one long path in which the different channels are periodically split off the main path in the desired fraction. This has the net result of requiring much less space on-chip for comparable optical path differences.
In traditional AWG, there are multiple parallel optical paths, each with a different engineered path-length. For high resolution, you want many different parallel paths and large differences in path length between the paths. To design this on a photonics chip requires significant area. The serial AWG creates a single path, equivalent to the longest path in the parallel AWG and split off fractions of the optical signal at various points along the way to create the equivalent path lengths. Serial Arrayed Waveguide Grating re-uses the same path instead of needing independent parallel paths.

Tunable Multi-Tone, Multi-Band, High-Frequency Synthesizer
Glenn's revolutionary new multi-tone, high-frequency synthesizer can enable a major upgrade in the design of high data rate, wide-band satellite communications links, in addition to the study of atmospheric effects. Conventional single-frequency beacon transmitters have a major limitation: they must assume that atmospheric attenuation and group delay effects are constant at all frequencies across the band of interest. Glenn's synthesizer overcomes this limitation by enabling measurements to be made at multiple frequencies across the entire multi-GHz wide frequency, providing much more accurate and actionable readings.
This novel synthesizer consists of a solid-state frequency comb or harmonic generator that uses step-recovery semiconductor diodes to generate a broad range of evenly spaced harmonic frequencies, which are coherent and tunable over a wide frequency range. These harmonics are then filtered by a tunable bandpass filter and amplified to the necessary power level by a tunable millimeter-wave power amplifier. Next, the amplified signals are transmitted as beacon signals from a satellite to a ground receiving station. By measuring the relative signal strength and phase at ground sites the atmospheric induced effects can be determined, enabling scientists to gather essential climate data on hurricanes and climate change. In addition, the synthesizer can serve as a wideband source in place of a satellite transponder, making it easier to downlink high volumes of collected data to the scientific community. Glenn's synthesizer enables a beacon transmitter that, from the economical CubeSat platform, offers simultaneous, fast, and more accurate wideband transmission from space through the Earth's atmosphere than has ever been possible before.