Carbon Fiber-Carbon Nanotube Yarn Hybrid Reinforcement
Materials and Coatings
Carbon Fiber-Carbon Nanotube Yarn Hybrid Reinforcement (LEW-TOPS-154)
Triaxial Braid Material for Polymer Matrix Composites
Overview
Innovators at the NASA Glenn Research Center have developed a toughened hybrid reinforcement material made from carbon fiber and carbon nanotube (CNT) yarn for use in polymer matrix composites (PMCs). The new material improves toughness and damping properties of PMCs, enhancing impact resistance, fatigue life, and structural longevity. Historically, PMCs have provided insufficient toughness for some aerospace structures. Rubber toughening agents and thermoplastics are often employed to increase material toughness, but such additives increase viscosity of polymer resins and increase processing difficulty and cost. Other reinforcements like aramids exist, but also have inherent issues (e.g., Kevlar absorbs moisture which adds weight and is susceptible to degradation upon UV exposure). NASA's new carbon fiber-CNT yarn hybrid material displays excellent toughness and strength for reinforcing PMC materials while also mitigating issues associated with current hybrid reinforcements like rubber, thermoplastics, and aramids.
The Technology
NASA's new material is a toughened triaxial braid made from ductile carbon nanotube (CNT) yarn hybridized with carbon fiber, which is ultimately used as reinforcement material to make toughened polymer matrix composites. The CNT yarn component of the reinforcement is solely responsible for adding toughness, while the processes used to optimize the fiber braiding parameters and tensile properties of the carbon fiber-CNT yarn hybrid tow material determine the overall improvement in tensile strength for resin impregnated fiber tows. Bundles of continuous carbon nanotube yarns are combined with a similar format of carbon fiber, yielding an easily scalable process.
Advantages of the material include reduced cost by eliminating use of toughening agents, increased ability to conform to highly complex geometries, greater environmental stability compared to aramid fiber reinforcements such as Kevlar, and possibly decreased density. Many hybrid reinforcements exhibit interfacial compatibility issues, which could lead to premature failure via crack propagation at the polymer matrix interface. In contrast, chemical similarities between the CNT yarn and carbon fiber constituents impart NASA's hybrid reinforcement material with excellent interfacial compatibility.
Potential applications include aerospace components, composite pressure vessels, wind turbine blades, automotive components, prosthetics, sporting equipment, construction reinforcement material, and other use-cases where strength-to-weight ratio is of utmost importance.
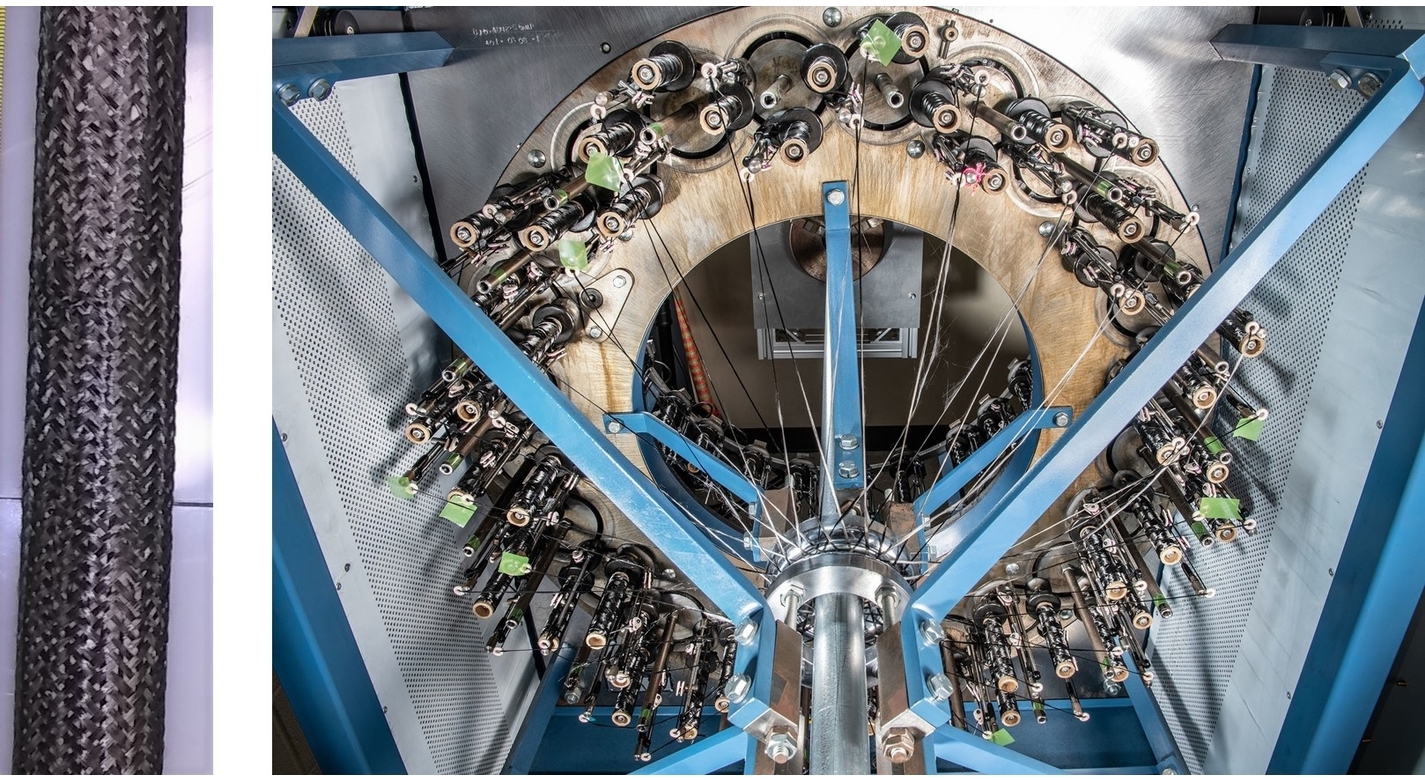
Benefits
- Eliminates need for rubber toughening agents or thermoplastics in PMC resins: High toughness can be achieved solely through the reinforcement material, enabling simpler processing (due to reduced resin viscosity) and reduced cost
- Ability to conform to complex geometries: Brittleness of carbon fiber reinforcement materials can be problematic for applications involving load bearing or complex geometries; this hybrid material displays a more ideal balance of stiffness and toughness
- Environmental stability: Not sensitive to UV exposure, unlike aramid fiber reinforcements (e.g., Kevlar)
- Mitigation of interfacial compatibility issues encountered by other hybrid reinforcements: Chemical similarities between reinforcement constituents alleviate interfacial mismatch that leads to premature material failure
Applications
- Aerospace and Aviation: aircraft components
- Automotive: PMC auto parts
- High-performance sports: advanced equipment (e.g., football helmets, hockey sticks, etc.)
- Medical devices: prosthetics
- Oil and Gas: high pressure gas storage vessels
- Power: wind turbine blades
- Unmanned Vehicles: drone components
Technology Details
Materials and Coatings
LEW-TOPS-154
LEW-19669-1
"Multifunctional Polymers and Composites for Aerospace Applications," Williams, Tiffany S., July 21, 2019, https://ntrs.nasa.gov/citations/20190032022.
Tags:
|
Similar Results

Vertically Aligned Carbon Nanotubes
Formation of the inventive polymer composite matrix begins by growing carbon nanotubes directly on a veil substrate. The carbon nanotubes are grown from both sides of a non woven carbon fiber mat. The carbon nanotubes can be single or multi walled and can be grown to predetermined lengths. The veiled substrate is positioned between carbon fiber/ polymer prepreg layers such that the carbon nanotubes protrude into the reinforcement layers. The polymer composite matrix formed following curing of the resin exhibits improved interlaminar strength, fracture toughness and impact resistance. Because of the thinness of the veil layer, electricity can pass from conductive carbon nanotubes on one side of the veil to conductive carbon nanotubes on the other side of the veil. Electricity can also pass between two veils intercalated into the same reinforcement layer when the length of the nanotubes is sufficiently long enough to provide overlap within the reinforcement layers.

Conductive Polymer/Carbon Nanotube Structural Materials and Methods for Making Same
Carbon nanotubes (CNTs) show promise for multifunctional materials for a range of applications due to their outstanding combination of mechanical, electrical and thermal properties. However, these promising mechanical properties have not translated well to CNT nanocomposites fabricated by conventional methods due to the weak load transfer between tubes or tube bundles.
In this invention, the carbon nanotube forms such as sheets and yarns were modified by in-situ polymerization with polyaniline, a -conjugated conductive polymer. The resulting CNT nanocomposites were subsequently post-processed to improve mechanical properties by hot pressing and carbonization. A significant improvement of mechanical properties of the polyaniline/carbon nanotube nanocomposites was achieved through a combination of stretching, polymerization, hot pressing, and carbonization.

Conductive Carbon Fiber Polymer Composite
The new composite developed by NASA incorporates PGS and CNTs to enhance its thermal conductivity while preserving the mechanical properties of the underlying carbon fiber polymer composite. NASA has also improved the composite manufacturing process to ensure better thermal conductivity not only on the surface, but also through the thickness of the material. This was achieved by adding perforations that enable the additives to spread through the composite.
The process for developing this innovative, highly thermally conductive hybrid carbon fiber polymer composite involves several steps. Firstly, a CNT-doped polymer resin is prepared to improve the matrix's thermal conductivity, which is then infused into a carbon fiber fabric. Secondly, PGS is treated to enhance its mechanical interface with the composite. Thirdly, perforation is done on the pyrolytic graphite sheet to improve the thermal conductivity through the thickness of the material by allowing CNT-doped resin to flow and better interlaminar mechanical strength. Finally, the layup of PGS and CNT-CF polymer is optimized.
Initial testing of the composite has shown significant increases in thermal conductivity compared to typical carbon fiber composites, with a more than tenfold increase. The composite also has higher thermal conductivity than aluminum alloys, with more than twice the thermal conductivity of the Aluminum 6061 typically used in the aerospace industry. For this new material, NASA has completed a proof-of-concept demonstration and work continues to use the material in a heat exchanger system and further characterize the properties including longevity and radiation impact analysis.

Dispersion of Carbon Nanotubes in Polymers
The technology portfolio spans several methods for dispersion and processing of CNTs in polymer resins and composites. CNT/resin systems with high dispersion and long-term stability are provided by three general approaches. One method relies on mechanical dispersion by sonication simultaneous with partial polymerization to increase the resin viscosity to maintain dispersion and enable further polymer processing of the CNT blend into films and other articles. Another approach relies on what is termed donor acceptor bonding, which essentially is a dipole bond created on the CNT/resin interface to maintain dispersion and stability of the CNT/resin blend. This dispersion method also provides advantages in mechanical properties of processed composites due to the interface characteristics. A range of polymer types can be used, including polymethyl methacrylate, polyimide, polyethylene, and others.
An additional dry blending approach provides advantages for a variety of
thermoplastic and thermoset systems. Use of ball mill mixing achieves effective
blending and dispersion of the CNT, even at high loadings. Further processing steps
using injection molding or similar melt processing methods have yielded CNT/
polymer composites with a range of useful electronic, optical, and mechanical
properties.

Low Creep, Low Relaxation Fiber-Reinforced Polymer Composites
NASA used three strategies to develop a family of modified epoxy resins for use in an improved composite layup configuration. By tailoring molecular structures, incorporating secondary additives, and adjusting composite architecture, NASA has produced fiber-reinforced polymer composites that suppress viscoelastic creep and relaxation. Specifically the three strategies were:
(1) Controlling the cross-linking density using reactive functional groups and selecting appropriate monomers with stoichiometry adjustments. A higher stoichiometric ratio is responsible for reduced relaxation compared to a lower ratio (resulting in 26% relaxation after 1 year vs. 49%).
(2) Increase the steric hindrance by reducing free volume and enhancing intermolecular interaction. This involved the preparation of two different reactive low molecular weight oligomers The best epoxy resin created was from the VCD/HAA additives with 15-17% relaxation; DEGBA/HAA had 22-25% relaxation.
(3) Optimizing different carbon fiber layup configurations for woven structures made into a highly stiff boom, highly flexible boom, a small size boom, and a large size boom application. The best configuration was that of a highly stiff boom with 4-5% relaxation. Other carbon fiber layup configurations had 16-30% relaxation.
As shown in the figure below, NASA tested the relaxed composite using an accelerated test with variable temperatures.