Adaptive Thermal Management System
materials and coatings
Adaptive Thermal Management System (KSC-TOPS-44)
A unique way to autonomously regulate the temperature of a structure or vessel
Overview
NASA Kennedy Space Center seeks partners interested in the commercial application of the Adaptive Thermal Management System (ATMS). Developed at the John F. Kennedy Space Center, the ATMS provides a way to regulate heat transfer and enable thermal management between two opposing surfaces in either direction. The system has the capability to adapt to provide conductive or insulative functionality depending on environmental conditions or applied stimuli. The ATMS can be designed for use in manufacturing, storage vessels, fluid transfer, aerospace and building architectures, and many other applications to reduce unwanted heat transfer, lower energy usage, or maintain environments at a specific temperature. The ATMS is part of NASAs technology transfer program, which seeks to promote the commercial use of NASA-developed technologies.
The Technology
Efficient thermal management has long been an issue in both commercial systems and in the extreme environments of space. In space exploration and habitation, significant challenges are experienced in providing fluid support systems such as cryogenic storage, life support, and habitats; or thermal control systems for launch vehicle protection, environmental heat management, or electronic instruments. Furthermore, these systems operate in dynamic, transient modes and often under extremes of temperature or pressure. The current technical requirements associated with the thermal management of these systems result in control issues as well as significant life-cycle costs.
To combat these issues, the Adaptive Thermal Management System (ATMS) was developed to help provide the capability for tanks, structural walls, or composite substrate materials to switch functionality (conductive or insulative) depending on environmental conditions or applied stimuli. As a result, the ATMS provides the ability to adapt between both heating and cooling modes within a single system. For example, shape memory alloy (SMA) elements are used to actuate at certain design temperatures to create a conductive bridge between two metal plates allowing broad-area heat rejection from the hotter surface. Upon cooling to the lower design set-point, the SMA elements return to their original shapes, thereby breaking the conductive path and returning the system to its overall insulative state.
This technology has the potential to be applied to any system that would have the need for a self-regulating thermal management system that allows for heat transfer from one side to another.
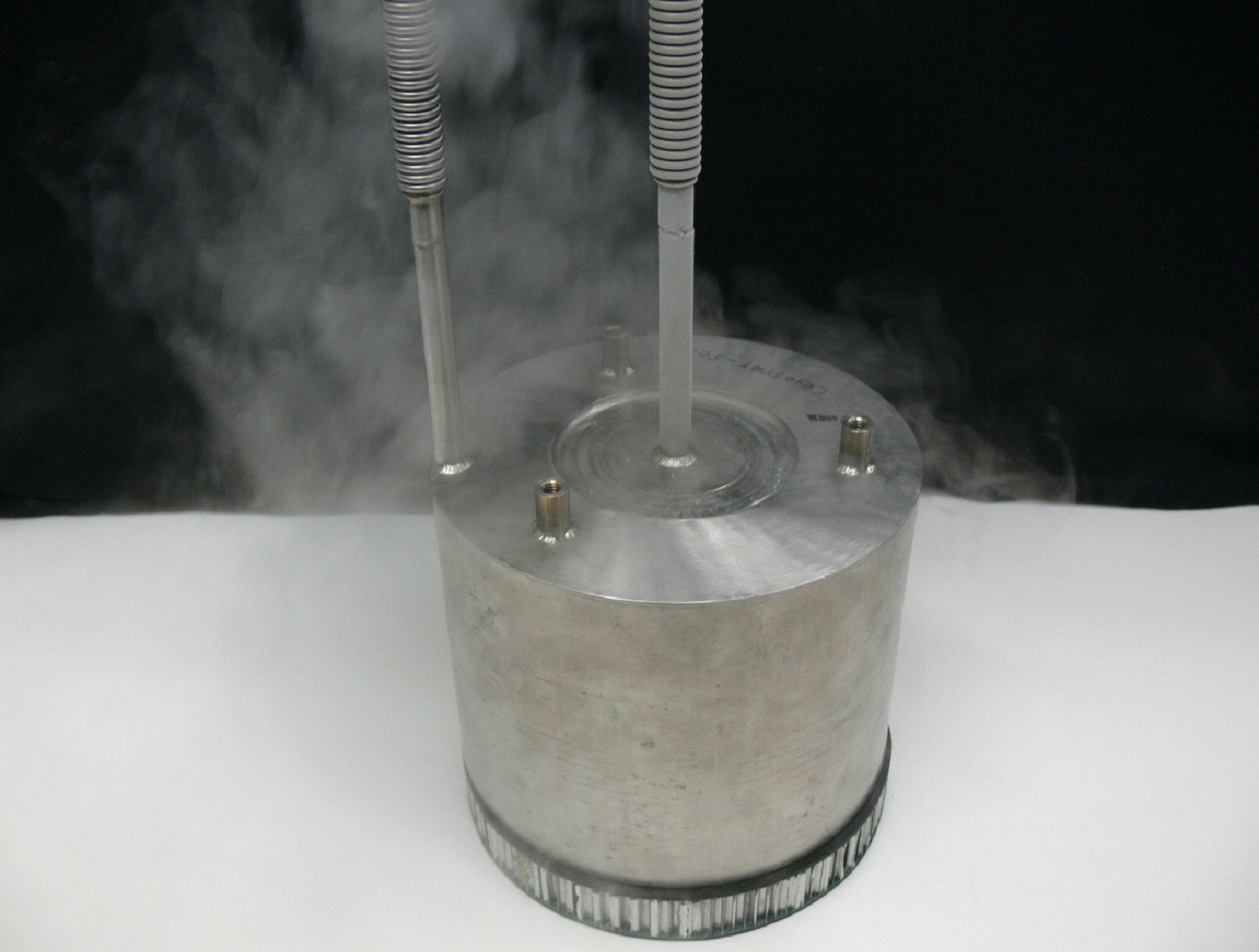
Benefits
- The system can be applied to broad planar areas for controlling heat transfer between two materials. It enables thermal conduction to provide heat dissipation to adjacent spaces.
- ATMS has the capability of switching functionality between conductive and insulative
- Passive system with no external activation required the system automatically reacts to environmental conditions
- Can be embedded into the designs of new systems or added to existing systems
- Flexibility in architectural design could allow for operation in a broad temperature range (from -150 C to 180 C)
- Energy Savings Since no mechanical or electrical activation is required, the system can autonomously regulate the temperature of a structure or vessel.
Applications
- Manufacturing Process Equipment
- Storage Vessels & Fluid Transfer
- Architecture/Housing and Habitats
- Commercial Building Envelopes
- Refrigerated Transport
- Aerospace Launch Vehicles and Tank Walls
- Power Plant Vessels
- Medical Imaging Equipment
- Airframes and Aircraft Integrated Structures
- Computers and Data Server Farms
Tags:
|
Similar Results

Ball Valve Assembly Yields Linear Flowrate Control
Prototype thermal control valves for the next generation spacesuit were challenged in maintaining precise thermal control, so engineers created a design that functions like a traditional ball valve but added tapered-valley contours to the ball that yields a variable orifice which is more predictable at controlling flow.
The key differences between the TCBV and traditional v-channel ball valves are that this technology has one inlet and two outlets allowing the split-flow of fluids whereas traditional v-channel valves only have one inlet and one outlet. Additionally, traditional v-channel ball valves don’t enable the full flow rate of a given system while this technology does.
The ball valve is held in place within the TCBV using two PTFE seats compressed by spring-loaded side plates. The hole in the middle of the ball valve and adjoining tapered valleys mate with the PTFE seats to create varying sized orifices depending on valve position. Specially designed O-ring seals surrounding the ball valve assembly allow the seats to move within the pocket while preventing internal leakage.
In this technology’s spacesuit application, coolant is fed to the ported ball valve where the coolant is apportioned to each valve housing exit either primarily feeding the cooling and ventilation garment or the bypass circuit back to the spacesuit’s thermal cooling system. The apportionment is determined by the astronaut’s manual valve adjustment or automatically by the suit.

3D-Printed Injector for Cryogenic Fluid Management
NASA's TVS Augmented Injector includes an internal heat exchanger, a fluid injector spray head, and an external surface condensation heat exchanger - all combined with multiple intertwined flow paths containing liquid, two-phase, and gaseous working fluid. The TVS provides a source of coolant to the injector, which chills the incoming fluid flow. This cooled flow promotes condensation of the tank ullage dropping pressure and maintains incoming fluid flow. The system eliminates the potential for a stalled fill condition and reduces tank pressure during cryogenic fluid transfer. During fill operations, the tank vent can be closed early in the process before fluid is introduced, and, in some cases, the tank vent may not even need to be opened. Furthermore, the TVS Augmented Injector can remove sufficient thermal energy to reach a 100% liquid level in the receiver tank. A cryo-cooler can be used in place the TVS flow circuit for a zero-loss system. The TVS Augmented Injector couples internal fluid flow cooling and external surface ullage gas condensation into a single, compact package that can be mounted to small tank flanges for minimal impact insertion into any vessel. The injector is printed as one part using additive manufacturing, resulting in part count reduction, improved reproducibility, shorter lead times, and reduced cost compared to conventional approaches.
The injector may be of particular interest in applications where cryogenic fluid is expensive, fluid loss through vents is problematic, and/or achieving high filling levels would be helpful. The injector can benefit typical cryogenic fluid transfer between containers or, alternatively, can serve as a tank pressure control device for long-term storage using a fluid recirculation system that pumps fluid through the injector and sprays cooled liquid back into the tank. Additionally, where ISRU processes are employed, the injector can be used to liquefy incoming propellant streams.

Macroflash (Cup Cryostat)
Advances in new polymers and composites along with growing industrial needs in below-ambient temperature applications have brought about the Macroflash development. Accurate thermal performance information, including effective thermal conductivity data, are needed under relevant end-use conditions. The Macroflash is a practical tool for basic testing of common materials or research evaluation of advanced materials/systems.
The Macroflash can test solids, foams, or powders that are homogeneous or layered in composition. Test specimens are typically 75mm in diameter and 6mm in thickness. The cold side is maintained by liquid nitrogen at 77 K while a heater disk maintains a steady warm-side temperature from ambient up to 373 K. The steady boiloff of the liquid nitrogen provides a direct measure of the heat energy transferred through the thickness of the test specimen. Nitrogen or other gas is supplied to the instrument to establish a stable, moisture-free, ambient pressure environment. Different compression loading levels can also be conveniently applied to the test specimen as needed for accurate, field-representative thermal performance data. The Macroflash is calibrated from approximately 10 mW/m-K to 800 mW/m-K using well-characterized materials.
Feedthrough for Severe Environments and Temperatures
Space and ground launch support related hardware often operate under extreme pressure, temperature, and corrosive conditions. When dealing with this type of equipment, it is frequently necessary to run wiring, tubes, or fibers through a barrier separating one process from another with one or both operating in extreme environments. Feedthroughs used to route the wiring, tubes, or fibers through these barriers must meet stringent sealing and leak tightness requirements.
This affordable NASA feedthrough meets or exceeds all sealing and leak requirements utilizing easy-to-assemble commercial-off-the-shelf hardware with no special tooling. The feedthrough is a fully reconfigurable design; however, it can also be produced as a permanent device. Thermal cycling and helium mass spectrometer leak testing under extreme conditions of full cryogenic temperatures and high vacuum have proven the sealing capability of this feedthrough with or without potting (epoxy fill) on the ends. Packing material disks used in the construction of the device can be replaced as needed for rebuilding a given feedthrough for another job or a different set of feeds if potting is not used for the original feedthrough build. (Potting on one or both sides of the sleeve provides double or triple leak sealing protection). Variable Compression Ratio (VCR) connectors were adapted for the pressure seal on the feedthrough; however, any commercial connector can be similarly adapted. The design can easily be scaled up to larger (2" diameter) and even very large (12" or more) sizes.

Thermal Management for Aircraft Propulsion Systems
Aircraft thermal management systems typically comprise over half the mass associated with full electric power propulsion systems, with significant negative impact on fuel efficiency. In addition, the traditional method of using jet fuel to cool aircraft generators does not provide enough cooling for use in flight-weight cryogenic systems. Lastly, the much higher bus voltages required for flight-weight systems (4.5 kV vs. 270 V) introduce additional spark-ignition hazards associated with alternative cryogenic cooling fuels, including liquid methane or liquid hydrogen. The Glenn flight-weight thermal management system addresses all of these problems by using the considerable waste heat energy from turbogenerators to create a pressure wave thermoacoustically. This wave can then be delivered quietly and efficiently via routed ductwork to hollow pulse-tube coolers located near any component in the aircraft that requires cooling. The tubes can be fabricated in any length and can be curved to fit any space. This technology also allows waste heat energy to be used in at least four ways: 1) the waste heat energy can drive a thermoacoustics-based ambient or cryogenic heat pump; 2) it can be channeled directly into a thermoacoustic engine that generates power; 3) it can convectively preheat the fuel/ or air supplied to the aircraft engine; 4) it can drive a pulse-tube generator providing power. The delivered thermoacoustic power can provide cabin cooling as well as ambient/cryogenic cooling of converter, cables, and motors. In addition, this power can be converted to local electric power through the use of a transducer (such as a linear alternator) or piezoelectrics. Further, the efficient thermal management system enables the size, mass, and resultant cost of the radiating fins to be reduced. Glenn's system offers an efficient method of cooling next-generation flight-weight electric aircraft with significant benefits for fuel efficiency and safety.