Multi-Layer Nuclear Thermionic Avalanche Cell
power generation and storage
Multi-Layer Nuclear Thermionic Avalanche Cell (LAR-TOPS-335)
High Power Density Power Source for Small to Large Scale Devices
Overview
Innovators at the NASA Langley Research Center (LaRC) have developed the Multi-Layer Nuclear Thermionic Avalanche Cell (NTAC), a novel electrical generator which transforms nuclear gamma-ray photon energy directly to electric power by liberating intra-band atomic inner shell electrons. The invention consists of several NTAC layers arranged in a radially concentric series separated by a vacuum gap space. A large number of electrons liberated within the emitter material are emitted from the surface, which has a tightly spaced array of nanometer-scale emitter points. Liberated electrons go across the vacuum gap and arrive at the collector to efficiently convert energy derived from radioactive materials into usable electricity. The device provides a compact, reliable, and continuous electrical source with high power density capable of long-life operation without refueling. The Multi-Layer NTAC is based on previous work at NASA LaRC in which a single emitter device captured high energy photons; use of a multilayer structure greatly improves the performance of the electrical generator.
The Technology
The Multi-Layer NTAC is comprised of a gamma-ray source and various layers of emitters, collectors, and insulators. Ideal emitter materials include elements with high atomic numbers, while ideal collector and insulator materials include elements with low atomic numbers. A high-energy gamma-ray (tens of keV to MeV) is used to liberate a large number of intra-band, inner-shell electrons from atoms within the emitter material for power generation through the primary interactions of photoelectric, Compton scattering, photonuclear, and electron/positron pair production processes. Secondary and tertiary electrons are liberated in the avalanche process as well. If a power conversion process effectively utilizes all liberated electrons in an avalanche mode through a power conversion circuit, the power output is drastically increased. Because power conversion is determined by the absorption rate of high energy photons, increasing power output requires either thicker collector material or a sufficient number of layer structures to capture the high energy photons, leaving no liberated electrons escaping (i.e., minimizing the leak of radioactive rays). The selection of materials, the thicknesses of the emitter, collector, and insulator, as well as the number of NTAC layers required are all determined by the energy of photon source. The thermal energy from radioactive decay can also be converted to electricity using a thermoelectric device to further increase power output. The Multi-Layer NTAC technology can be manufactured using existing semiconductor fabrication technology and can be tailored for small-to-large scale power needs, including kilowatt and megawatt applications.
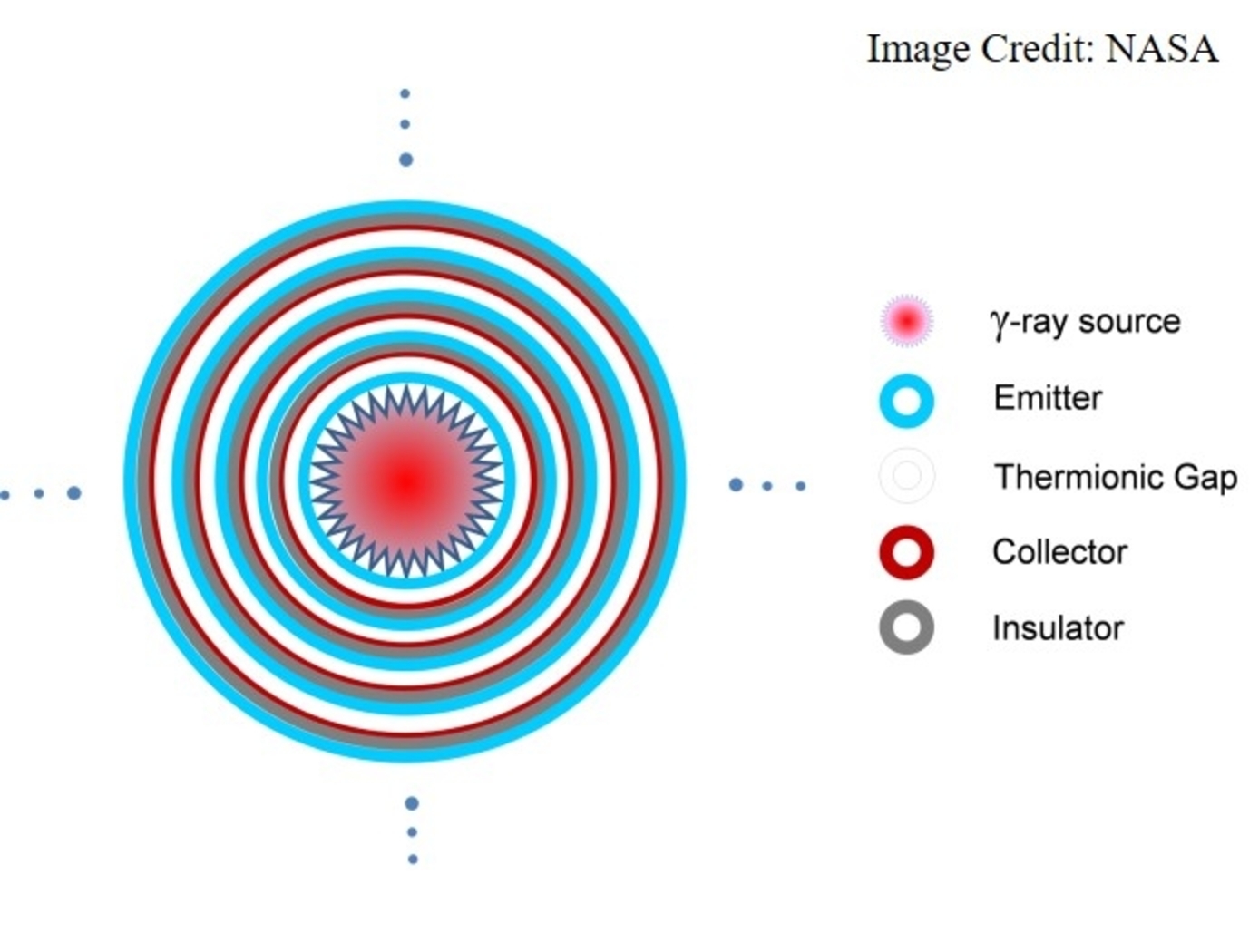
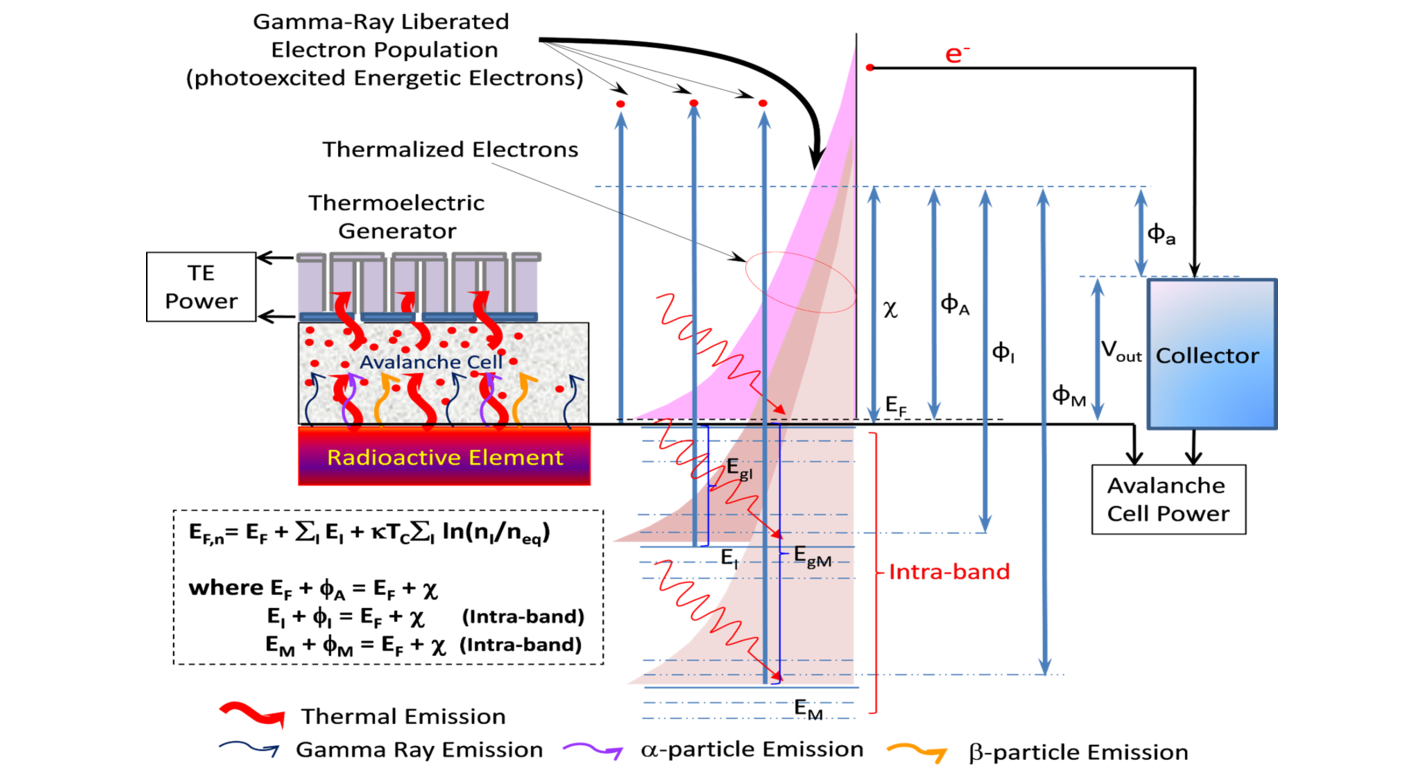
Benefits
- More simple and efficient than competing methods: thermionic emission is superior to radioisotope thermoelectric generation, with reported electron emission of 10-20% for single-layer NTAC versus 6-7% for TEG (multi-layer NTAC will double or triple the electron emission efficiency)
- Ease of manufacturing: The NTAC technology adapts well-established semiconductor manufacturing techniques, lowering the initial investment costs
- Compact size: the use of thin-film structures enables the device to be fabricated as small as a button cell
- Long-lasting power source: because the half-life of radioactive materials can be nearly a century, a single NTAC charge can run for decades without refueling
Applications
- Aerospace: power for systems on small satellites, drones, commercial airliners, spacecraft, etc.
- Automotive: electric vehicles
- Power: lightweight, compact, long-lived nuclear batteries
- Propulsion: deep space exploration, UAVs, electric aircraft, etc.
- Security: power for various security systems without dependence on the power grid
Technology Details
power generation and storage
LAR-TOPS-335
LAR-19253-1
LAR-18860-1
LAR-17981-1
Tags:
|
Similar Results

Improved Efficiency in Nuclear Propulsion
Current nuclear propulsion technologies do not utilize the additional energy expelled as gamma ray radiation during nuclear fission. This results in the loss of 6.5% of the total fission energy, which could be used to improve propulsion capabilities. The NTAC Augmented Nuclear Electric Propulsion and/or Nuclear Thermal Propulsion design is more efficient than existing technology and captures an additional 6.5% (13.3 MeV) of radiation energy that is currently lost to radiation shielding. In this design the NTAC cell structure surrounds the TRISO (TRi-structural ISOtropic particle fuel) bead-filled rocket chamber, covering all sides and the top of the chamber, therefore capturing additional energy. This structure allows one-way energy flow to then be expelled through the bottom cavity as exhaust gas
This technology is lower weight and less elaborate than current radiation shielded-propulsion systems. Equipping nuclear spacecraft systems with the NTAC Augmented Nuclear Electric Propulsion and/or Nuclear Thermal Propulsion design could reduce travel time to Mars by 50%. It has lower radar signatures compared to solar panels and could be used for systems that need to fly undetected, such as Earth observing satellites. Additionally, it has a lower noise output, which could have applications in reducing noise from submarine and aircraft carrier power systems.

Nuclear Thermionic and Thermoelectric Energy System
The NASA patented NTAC-TE system is a promising technology for power generation from radioactive isotopes that generate gamma rays. This will reduce the mass of unusable radioactive waste products, as NTAC-TE can use what is normally waste and harmful in order to generate electric power.
The patented integration of Cobalt-59 into fission process, which will be transmuted into Co-60, with NTAC-TE elevates the efficiency well beyond current energy harvesting methodologies considering NTAC-TE can also harness energy from Cesium-137. The gamma radiation energies from these three sources combined together are 7 MeV from the prompt fission reaction, 0.6617 MeV from the Cesium by-product of the fission process, and 1.3325 MeV from Cobalt.

Portable Compact Thermionic Power Cell
This compact thermionic cell (CTI) technology can be manufactured efficiently and economically using existing semiconductor fabrication technology. Its design consists of a top electron collector, separated by a vacuum gap from an electron emitter adjacent to the heat source, a thin plate of 238Pu enclosed by a thin-film insulator to protect the emitter and collector layers from overheating by the 238Pu. For a smart phone battery size, the invented compact thermionic (CTI) cell requires about 5 g maximum of 238Pu. Such small quantities are more readily available and producible, and could be reused for recycling when the CTI cell is dismantled. The emitter surface is topologically modified to have array of spikes, achievable using current semiconductor microfabrication technology. Various other geometries of emitter plates may also be used, such as an array of ridges. The smaller the emitter tips, the higher the voltage concentration.

Low-Power Charged Particle Detector
Conventional scintillation radiation detectors make use of materials that emit light when hit by ionizing radiation. These large, bulky scintillators range anywhere from 6 inches to 6 feet in size. They are attached to glass photomultiplier tubes (PMTs), which are fragile, require high voltages, and are extremely sensitive to temperature changes. Conventional detectors also include a wave shifter (a material with a dopant to re-emit the scintillator light to match the sensitivity of the PMT or photodiode) which reduces efficiency and introduces unwanted weight and bulk. Glenn's particle counter overcomes these challenges. A prototype was constructed from off-the-shelf components, and features a small scintillator (less than six inches long) and a low-voltage, UV-sensitive, wide-bandgap photodiode as the detector. Careful matching of the properties of the light emitted from the scintillator, and tailoring the sensitivity of the photodiode eliminates the need for a PMT and a wave shifter, not only saving space, weight, and power but also eliminating potential failure modes. By using wide-bandgap detectors, it can operate in changing temperature, vibration, pressure, and gravity conditions without need for a temperature compensation system. Built from solid-state components, Glenn's device is compact and robust.

Fine-Grained Targets
The fine-grained targets are a matrix of a prismatic edge natural flake graphite that produces strong structural pi bonds when pressed at room temperature. The targets have an approximate grain size of 2 microns. The graphite provides a carbon source that is combined with a metal catalyst made of powder forge nickel and powder forge cobalt. Previously used targets involved pressing and binding targets with Dylon carbon cement. These particles had an approximate 200-micron particle size, which led to large regions of uncatalyzed target. The new metal powders are small (~0.5 microns), highly dispersible, and two orders of magnitude less expensive. The powders are mixed in appropriate quantities and subjected to low-energy ball-milling to
ensure mingling. Targets undergo additional preparatory steps, including high pressure processing. The targets are then subjected to a series of ultrafast infrared laser light pulses at a high repetition rate to vaporize the layers of the spinning targets and create a plume of nanotubes.