More Reliable Doppler Lidar for Autonomous Navigation
sensors
More Reliable Doppler Lidar for Autonomous Navigation (LAR-TOPS-351)
Sensor algorithm salvages compromised lidar signal data
Overview
NASA pioneered Navigation Doppler Lidar (NDL) for precision navigation and executing well-controlled landings on surfaces like the moon. The lidar sensor utilizes Frequency Modulated Continuous Wave (FMCW) technique to determine the distance to the target and the velocity between the sensor and target. Specifically, homodone sensors obtain the changes in signal frequency between the received and reference frequencies for calculating both speed and distance. However, homodyne detection cannot provide any phase information. This is a problem because the current sensor cannot determine the sign (+/-) of the signal frequencies, resulting in false measurements of range and velocity. NASA has developed an operational prototype (TRL 6) of the method and algorithm that works with the receiver to correct the problem. Using a three-section waveform and an algorithm to resolve ambiguities in sign when the signal is compromised, the algorithm analyzes historical phase information to interpret the sign of the remaining frequencies and recover the phase information that contains valuable measurement information.
The Technology
The NDL uses homodyne detection to obtain changes in signal frequency caused by a target of interest. Frequency associated with each segment of the modulated waveform collected by the instrument is positive or negative, depending on the relative range and direction of motion between the NDL and the target. Homodyne detection offers a direct measurement of signal frequency changes however only the absolute values of the frequencies are measured, therefore additional information is necessary to determine positive or negative sign of the detected frequencies. The three segmented waveform, as opposed to conventional two-segmented ones, allows for resolving the frequency sign ambiguity. In a practical system, there are times when one or more of the three frequencies are not available during a measurement. For these cases, knowledge of the relative positions of the frequency sideband components is used to predict direction of the Doppler shift and sign, and thus make correct range and velocity measurements. This algorithm provides estimates to the sign of the intermediate frequencies. The instrument operates continuously in real time, producing independent range and velocity measurements by each line of sight used to take the measurement. In case of loss of one of the three frequencies, past measurements of range and velocity are used by the algorithm to provide estimates of the expected new range and velocity measurement. These estimates are obtained by applying an estimation filter to past measurements. These estimates are used during signal loss to reduce uncertainty in the sign of the frequencies measured once signals are re-established, and never to replace value of a measurement.
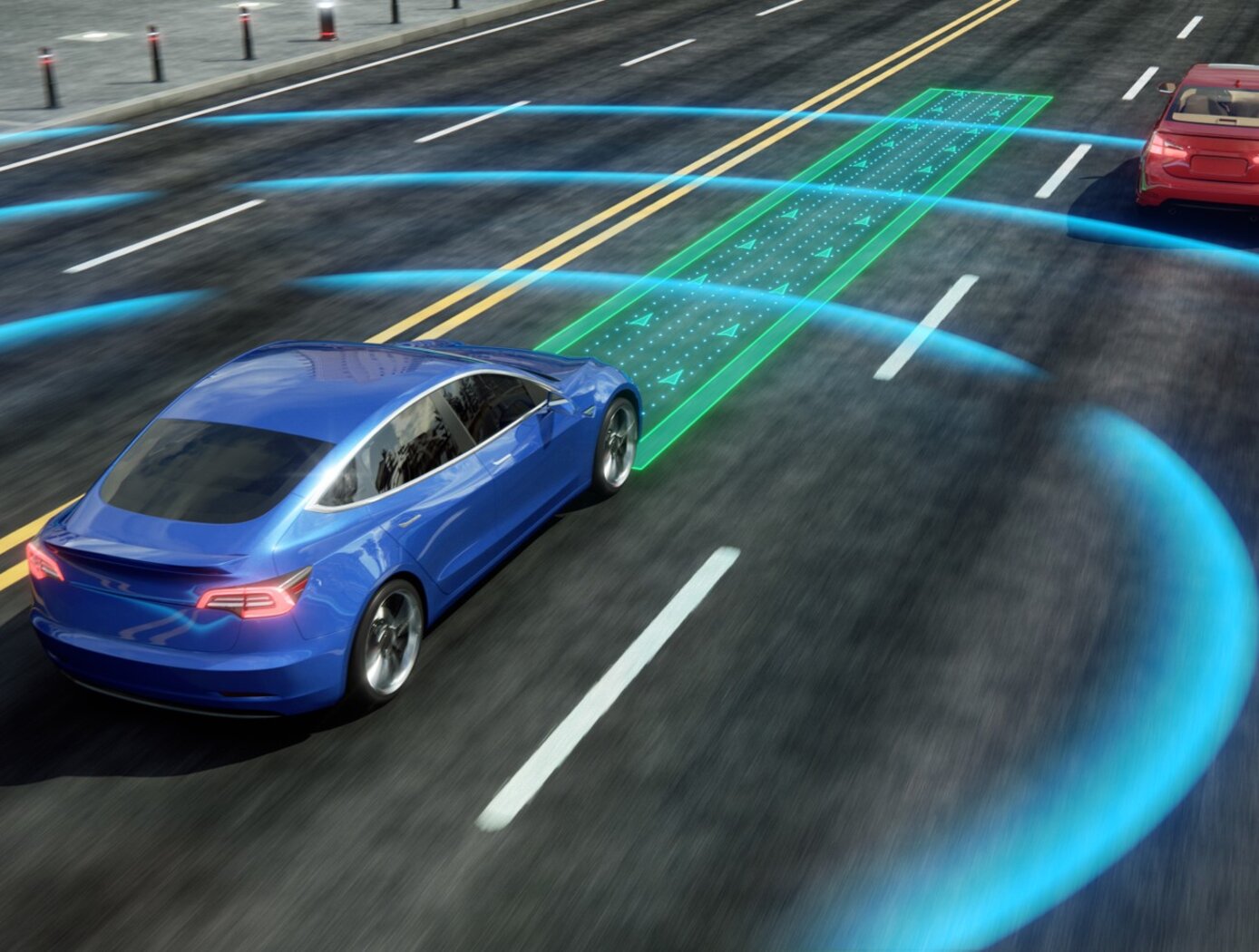
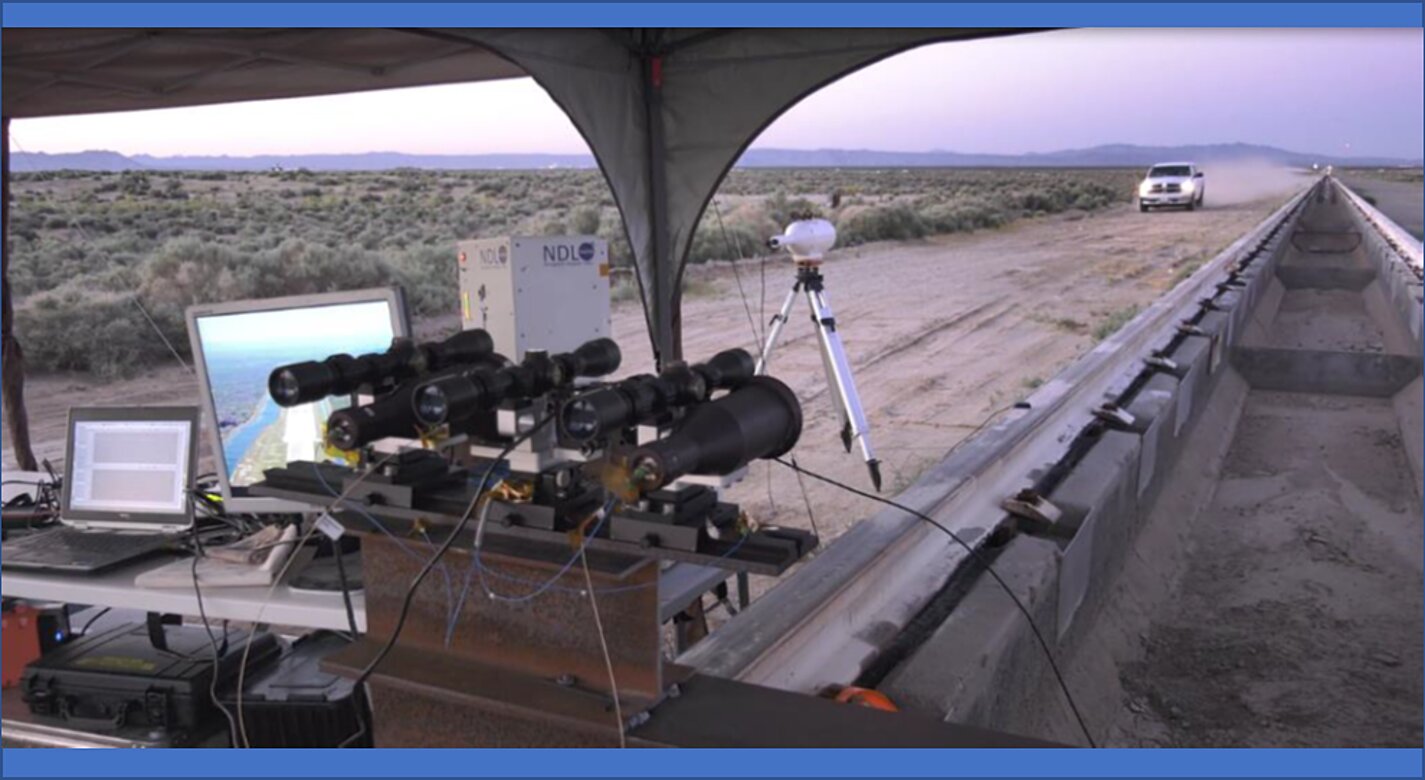
Benefits
- Increased reliability of lidar data: reduces ambiguities that are due to partial loss of a signal
- Easy implementation: algorithm works with current homodone sensors
- Optimal data use: recovers valuable information from partial data signals
- Improves safety and efficiency: reduces the chance of errors due to misread signals.
Applications
- Self-driving cars: provides both 3D range and Doppler (velocity) images of surroundings
- Aircraft Navigation: system allows navigation in GPS-denied environments
- General Automated Rendezvous and Docking: provides relative position, approach velocity, and relative orientation and attitude of the docking port.
- Space: NDL can enable precision navigation to the desired landing location for future large robotic and crewed landing missions,.
Technology Details
sensors
LAR-TOPS-351
LAR-19687-1
Amzajerdian, Pierrottet, et. al. Navigation Doppler Lidar Performance at High Speed and Long Range. 2020 AIAA SciTech Forum. April 2020.
Farzin Amzajerdian, Glenn D. Hines, et al. Characterization of a Coherent Doppler Lidar for Operation Onboard Aerial and Space Vehicles. OSA Laser Congress September 29 - October 3, 2019.
Farzin Amzajerdian, Glenn D. Hines, et al. Characterization of a Coherent Doppler Lidar for Operation Onboard Aerial and Space Vehicles. OSA Laser Congress September 29 - October 3, 2019.
Tags:
|
|
Related Links:
|
Similar Results

Laser Linear Frequency Modulation System
For decades, frequency modulation has been used to generate chirps, the signals produced and interpreted by sonar and radar systems. Traditionally, a radio or microwave signal is transmitted toward the target and reflected back to a detector, which records the time elapsed and calculates the targets distance. Reflected signals can be heterodyned (combined) with output signals to determine the Doppler frequency shift and the target velocity. Accuracy of these systems can be enhanced by increasing the bandwidth of the chirp, but noise generated during heterodyning at high frequencies decreases the signal-to-noise ratio, increasing measurement error.
Previous attempts at laser frequency modulation that relied on adjusting the laser cavity length have resulted in only sine wave or imperfect triangle waveforms. Heterodyning of imperfect, non-linear waveforms or sine waveforms will significantly degrade the effective signal-to-noise ratio, making such systems impractical. In contrast, the current technology produces a single, high-frequency laser that is passed to an electro-optical modulator, which generates a series of harmonics. This range of frequencies is then passed through a band-pass optical filter so the desired harmonic frequency can be
isolated and directed toward the target. By modulating the electrical signal applied to the electro-optical modulator, a near perfect triangular waveform laser beam can be produced. Transmission and detection of this highly linear triangular waveform facilitates optical heterodyning for the calculation of precise frequency and phase shifts between the output and reflected signals with a high signal-to-noise ratio. By combining this information with the time elapsed, the location and velocity of the target can be determined to within 1 mm or 1 mm/s.

Recirculating Advanced Coupled-cavity Etalon Receiver (RACER)
Advanced Coupled-cavity Etalon (ACE) significantly improves both in-band transmission and out-of-band rejection. In some cases, 12% more light is transmitted inside the passband and >3x more light is rejected outside the passband. Incorporating ACE into the recirculating etalon receiver (RER) improves performance significantly. ACE increases the wavelength resolution and enables closer channel spacing resulting in a very efficient, high resolution spectrometer. RACER has both high resolution and a high photon efficiency which allows flexibility for trading different combinations of reduced cross-talk and closer channel spacing.

Pulsed 2-Micron Laser Transmitter
The new NASA LaRC Pulsed 2-Micron Laser Transmitter for Coherent 3-D Doppler Wind Lidar Systems is an innovative concept and architecture based on a Tm:Fiber laser end-pumped Ho:YAG laser transmitter. This transmitter meets the requirements for space-based coherent Doppler wind lidar while reducing the mission failure risks. A key advantage of this YAG based transmitter technology includes the fact that the design is based on mature and low-risk space-qualified YAG host crystal. The transmitter operates at a 2096 nm wavelength using Ho:YAG, resulting in high atmospheric transmission (>99%), versus a transmitter operating at 2053 nm using co- doped Tm:Ho:LuLiF, which suffers limited transmission (90%) due to water vapor interference. In-band pumping through Tm:Fiber pump Ho:YAG architecture offers lower quantum defect from 1908 to 2096 nm (9.1%) compared to traditionally used co-doped Tm:Ho:LuLiF of 792 to 2051 nm (61%). The transmitter has an efficient pump compared to LuLF, since YAG has 27% higher pump absorption and 52% lower reabsorption of the emitted 2-micron, resulting in higher efficiency and lower heat load. Being isotropic, YAG is amenable for spatial-hole burning mitigation which supports linear cavity architecture without compromising injection seeding quality. This attribute is important in designing a compact, stable, high seeding efficiency laser. A folded linear cavity for long pulse (>200 ns), transform limited line-width (2.2 MHz) and high beam quality (M2 = 1.04) - the most critical parameters for coherent detection - are easier to achieve using YAG compared to LuLF. Lower heat load results in high repetition rate (>300 Hz) operation, which allows higher probability of wind measurements through broken clouds, off clouds, and below clouds, thus reducing errors and increasing science data product quantity and quality.

Real-Time LiDAR Signal Processing FPGA Modules
The developed FPGA modules discern time-of-flight of laser pulses for LiDAR applications through the correlation of a Gaussian pulse with a discretely sampled waveform from the LiDAR receiver. For GRSSLi, up to eight cross-correlation engines were instantiated within a FPGA to process the discretely sampled transmit, receive pulses from the LiDAR receiver, and ultimately measure the time-of-flight of laser pulses at 20-picosecond resolution. Engine number is limited only by the resources within the FPGA fabric, and is configurable with a constant. Thus, potential time-of-flight measurement rates could go well beyond the 200-KHz mark required by GRSSLi. Additionally, the engines have been designed in an extremely efficient manner and utilize the least amount of FPGA resources possible.

3D Lidar for Autonomous Landing Site Selection
Aerial planetary exploration spacecraft require lightweight, compact, and low power sensing systems to enable successful landing operations. The Ocellus 3D lidar meets those criteria as well as being able to withstand harsh planetary environments. Further, the new tool is based on space-qualified components and lidar technology previously developed at NASA Goddard (i.e., the Kodiak 3D lidar) as shown in the figure below.
The Ocellus 3D lidar quickly scans a near infrared laser across a planetary surface, receives that signal, and translates it into a 3D point cloud. Using a laser source, fast scanning MEMS (micro-electromechanical system)-based mirrors, and NASA-developed processing electronics, the 3D point clouds are created and converted into elevations and images onboard the craft. At ~2 km altitudes, Ocellus acts as an altimeter and at altitudes below 200 m the tool produces images and terrain maps. The produced high resolution (centimeter-scale) elevations are used by the spacecraft to assess safe landing sites.
The Ocellus 3D lidar is applicable to planetary and lunar exploration by unmanned or crewed aerial vehicles and may be adapted for assisting in-space servicing, assembly, and manufacturing operations. Beyond exploratory space missions, the new compact 3D lidar may be used for aerial navigation in the defense or commercial space sectors. The Ocellus 3D lidar is available for patent licensing.