Improving VTOL Proprotor Stability
Mechanical and Fluid Systems
Improving VTOL Proprotor Stability (LAR-TOPS-374)
Adaptive control methodology provides tiltrotor aircraft improved operational boundary and vibration reduction
Overview
Vertical take-off and landing (VTOL) aircraft such as tiltrotors hold the promise to provide the next generation of air transport but are limited in their forward airspeeds due to complex, coupled rotor and wing dynamic instabilities. NASA Langley’s Advanced Generalized Predictive Control (AGPC) technology provides swashplate actuators with high-frequency control signals to stabilize dynamic instabilities allowing envelope expansion and to reduce vibration anywhere in the envelope. Compared to existing Generalized Predictive Control (GPC) systems, AGPC is self-adaptive and compares actual system behavior with that predicted by experimentally-derived system identification parameters. This methodology is unique in that modeling parameters are updated by observation of the operational controller and requires no or negligible excitation of the system in order for the adaptation to be successful.
The Technology
Proprotors on tiltrotor aircraft have complex aeroelastic properties, experiencing torsion, bending, and chord movement vibrational modes, in addition to whirl flutter dynamic instabilities. These dynamics can be stabilized by high-frequency swashplate adjustments to alter the incidence angle between the swashplate and the rotor shaft (cyclic control) and blade pitch (collective control). To make these high-speed adjustments while minimizing control inputs, generalized predictive control (GPC) algorithms predict future outputs based on previous system behavior. However, these algorithms are limited by the fact that tiltrotor systems can substantially change in orientation and airspeed during a normal flight regime, breaking system continuity for predictive modeling.
NASA’s Advanced GPC (AGPC) is a self-adaptive algorithm that overcomes these limitations by identifying system changes and adapting its predictive behavior as flight conditions change. If system vibration conditions deteriorate below a set threshold for a set time interval, the AGPC will incrementally update its model parameters to improve damping response. AGPC has shown significant performance enhancements over conventional GPC algorithms in comparative simulations based on an analytical model of NASA’s TiltRotor Aeroelastic Stability Testbed (TRAST). Research for Hardware-In-the-Loop testing and flight vehicle deployment is ongoing, and hover data show improved vibration reduction and stability performance using AGPC over other methods.
The example presented here is an application to tiltrotor aircraft for envelope expansion and vibration reduction. However, AGPC can be employed on many dynamic systems.
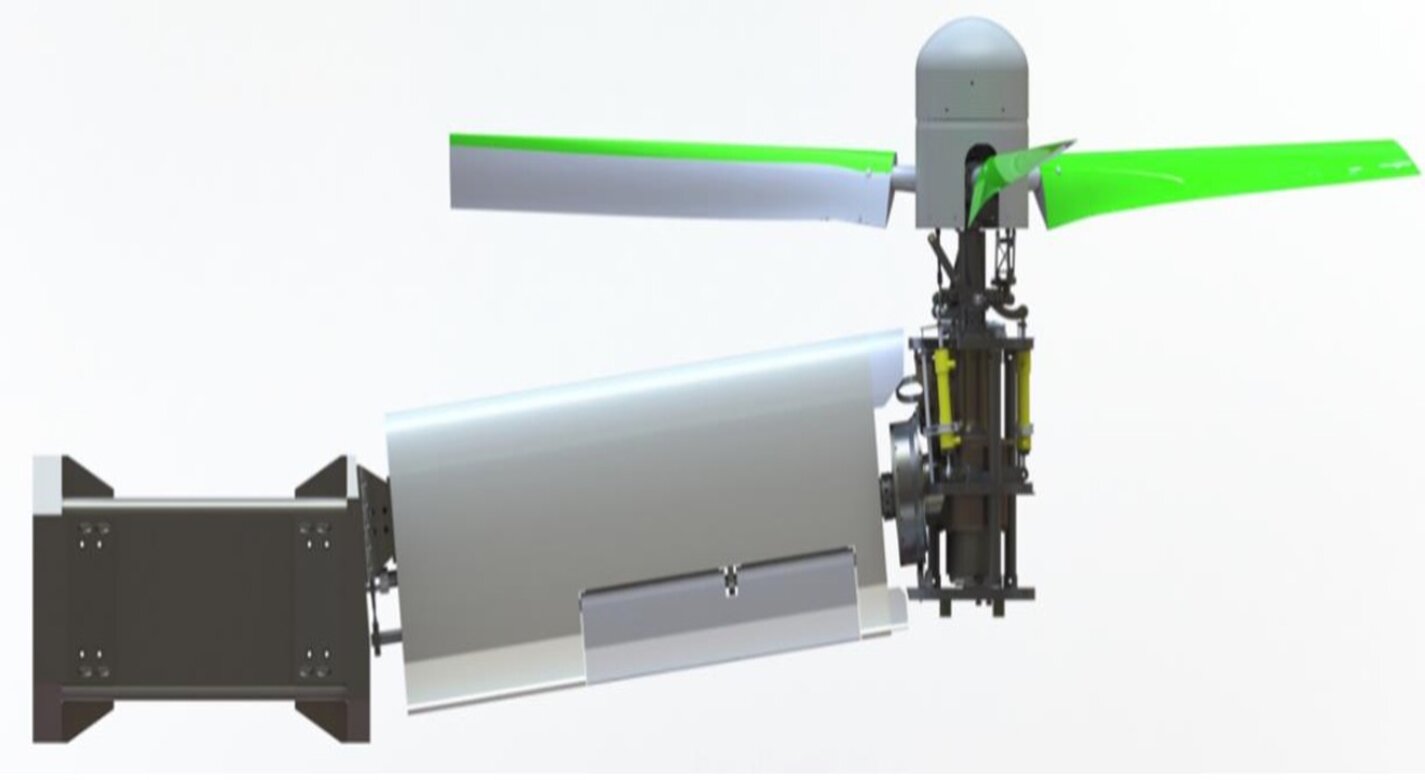
Benefits
- Active Ride Control: Self-adaptive control response optimizes damping performance even as flight conditions change.
- Faster Forward Flight Speeds: AGPC eliminates vibrations that would otherwise destabilize proprotors at high airspeeds.
- Ease of Implementation: No additional manual inputs are required to trigger control system corrections to match new flight conditions.
- Expanded Operational Boundaries: May provide faster gust attenuation and flutter suppression response times, improving craft airworthiness.
Applications
- Urban Air Mobility: Active Ride Control for Passenger Comfort and possible extension as flight controller for changing conditions
- Aerospace: Controllers for surface actuators or hydraulic systems
- Military: Rotorcraft & Payload Stabilization
- Other dynamic systems that employ active controls
Technology Details
Mechanical and Fluid Systems
LAR-TOPS-374
LAR-20145-1
“Generalized Predictive Control for Active Stability Augmentation and Vibration Reduction on an Aeroelastic Tiltrotor Model.” AIAA SciTech 2022 Forum Conference Paper, January 3, 2022.
"Advanced Generalized Predictive Control and its Application to Tiltrotor Aircraft for Stability Augmentation and Vibration Reduction." Dissertation, Old Dominion University, December 2022.
"Experimental Testing of Advanced Generalized Predictive Control for Stability Augmentation and Vibration Reduction of Tiltrotor Aircraft." Vertical Flight Society International, Forum Conference Paper, May 2023.
"Advanced Generalized Predictive Control and its Application to Tiltrotor Aircraft for Stability Augmentation and Vibration Reduction." Dissertation, Old Dominion University, December 2022.
"Experimental Testing of Advanced Generalized Predictive Control for Stability Augmentation and Vibration Reduction of Tiltrotor Aircraft." Vertical Flight Society International, Forum Conference Paper, May 2023.
Similar Results

Aerodynamically Actuated Thrust Vectoring Device
The thrust actuating device includes several innovations in the aerodynamically stable tilt actuation of propellers, propeller pylons, jets, wings, and fuselages, collectively called propulsors. The propulsors rotate between hover and forward flight mode for a tilt-wing or tilt-rotor aircraft. A vehicle designed using this technology can transition from a hovering flight condition to a wing born flight condition with no mechanical actuation and can do so without complex control systems. This results in a reduction in system weight and complexity and produces a robust and naturally stable hovering aircraft with efficient forward flight modes.

Rapid Aero Modeling for Computational Experiments
RAM-C interfaces with computational software to provide test logic and manage a unique process that implements three main bodies of theory: (a) aircraft system identification (SID), (b) design of experiment (DOE), and (c) CFD. SID defines any number of alternative estimation methods that can be used effectively under the RAM-C process (e.g., machine learning techniques, regression, neural nets, fuzzy modeling, etc.). DOE provides a statistically rigorous, sequential approach that defines the test points required for a given model complexity. Typical DOE test points are optimized to reduce either estimation error or prediction error. CFD provides a large range of fidelity for estimating aircraft aerodynamic responses. In initial implementations, NASA researchers “wrapped” RAM-C around OVERFLOW, a NASA-developed high-fidelity CFD flow solver. Alternative computational software requiring less time and computational resources could be also utilized.
RAM-C generates reduced-order aerodynamic models of aircraft. The software process begins with the user entering a desired level of fidelity and a test configuration defined in terms appropriate for the computational code in use. One can think of the computational code (e.g., high-fidelity CFD flow solver) as the “test facility” with which RAM-C communicates with to guide the modeling process. RAM-C logic determines where data needs to be collected, when the mathematical model structure needs to increase in order, and when the models satisfy the desired level of fidelity.
RAM-C is an efficient, statistically rigorous, automated testing process that only collects data required to identify models that achieve user-defined levels of fidelity – streamlining the modeling process and saving computational resources and time. At NASA, the same Rapid Aero Modeling (RAM) concept has also been applied to other “test facilities” (e.g., wind tunnel test facilities in lieu of CFD software).

Outer Aileron Yaw Damper
Rudders have long served as the primary flight control surface as is pertains to aircraft yaw. Breaking this mold, NASA's SAW technology is a game-changing development in aircraft wing engineering that reduces rudder motion required to control aircraft. The benefits of reduced rudder dependency led NASA to develop the outer aileron yaw damper to further decrease or eliminate rudder dependency for aircraft using SAWs.
As mentioned, SAWs use shape memory alloy actuators to articulate the outer portion of the wing, effectively creating a movable wingtip. NASA's invention uses an outer aileron located on the wingtips, which is driven (along with the inner ailerons) by a novel control algorithm. The control algorithm, taking into account the wingtip positions, manipulates the outer ailerons to achieve the desired yaw rate. At the same time, it positions the inner ailerons to counter roll rate resulting from the outer aileron. In other words, the control algorithm calculates a control surface ratio (i.e., position of inboard aileron and outboard aileron) that produces desired yaw and roll accelerations.
The system can also be used to offset the existing rudder in current or future aircraft designs. A second part of NASAs novel outer aileron control algorithm modifies the aircrafts rudder loop gain in proportion to outer aileron usage. This allows the outer ailerons and rudder to work in tandem, while at the same time reducing rudder usage.
As a result of this NASA invention, required rudder usage can be reduced or eliminated for aircraft with SAWs. Consequently, the size of rudders and vertical tail structures can be reduced, which in turn reduces weight and parasitic drag. The result is an aircraft with increased performance and fuel efficiency.

Fixed Wing Angle eVTOL
While previous eVTOLs often require a near 90° wing tilt to position propellers in an optimal location to generate vertical force for takeoff, NASA has taken a very different approach. NASA's design instead uses a slight wing angle and large flaps designed to deflect slipstream generated by the propellers to create a net positive force in the vertical direction, all while preventing forward movement. This unique configuration allows for takeoff and landing operations without the need for near 90° wing tilt angles. After takeoff, the transition to forward flight only requires a slight change in attitude of the vehicle and retraction of the flaps. Similar solutions require large changes in attitude to accomplish this transition which is often undesirable, especially for air taxi operations that involve passengers.
Given the effectiveness of this configuration for generating upward force, the requirement for wing angle tilt has been reduced from near 90° to approximately 15° during takeoff. Further iterations may reduce this requirement even further to 0°. By eliminating the need for near 90° wing tilt, NASA's eVTOL design removes the need for mechanisms to perform active tilting of the wings or rotors, reducing system mass and thereby improving performance. Flaps represent the only components that require actuation for takeoff and landing operations.
Innovators at NASA leveraged the Langley Aerodrome 8 (LA-8), a modular testbed vehicle that allows for rapid prototyping and testing of eVTOLs with various configurations, to design and test this novel concept.

Improved Fixed-Wing Gust Load Alleviation Device
Gust loads may have detrimental impacts on flight including increased structural and aerodynamic loads, structural deformation, and decreased flight dynamic performance. This technology has been demonstrated to improve current gust load alleviation by use of a trailing-edge, free-floating surface control with a mass balance. Immediately upon impact, the inertial response of the mass balance shifts the center of gravity in front of the hinge line to develop an opposing aerodynamic force alleviating the load felt by the wing. This passive gust alleviation control covering 33% of the span of a cantilever wing was tested in NASA Langleys low speed wind tunnel and found to reduce wing response by 30%.
While ongoing experimental work with new laser sensing technologies is predicted to similarly reduce gust load, simplicity of design of the present invention may be advantageous for certification processes. Additionally, this passive technology may provide further gust alleviation upon extending the use of the control to the entire trailing edge of the wing or upon incorporation with current active gust alleviation systems.
Importantly, the technology can be easily incorporated into to the build of nearly all fixed wing aircrafts and pilot control can be maintained through a secondary trim tab. Though challenging to retrofit, passive gust alleviation could enable use of thinner, more efficient wings in new plane design.