Fine-Grained Targets
materials and coatings
Fine-Grained Targets (LAR-TOPS-67)
High-yield synthesis of carbon nanotubes via free electron laser ablation
Overview
NASA's Langley Research Center scientists originally developed a Free Electron Laser (FEL) Ablation technique to synthesize single-walled carbon nanotubes. FEL provides a vast improvement over other techniques employed to make carbon nanotubes. The nanotubes produced by FEL are very pure,
straight, homogenous, and defect-free. Most notable is that these nanotubes can be produced in much larger quantities than by other laser-based techniques. Now NASA scientists have further provided incremental improvement in this technology by creating a recipe and procedure for the inexpensive and simple production of fine-grained targets required for the synthesis of carbon nanotubes using FEL. Previously used targets employed metal powders that did not have a particularly small grain size or small size dispersibility. The targets used here are made of powder forge metals that are both small and dispersible. The targets facilitate a significant cost reduction in the supply of high-quality single-walled carbon nanotubes.
The Technology
The fine-grained targets are a matrix of a prismatic edge natural flake graphite that produces strong structural pi bonds when pressed at room temperature. The targets have an approximate grain size of 2 microns. The graphite provides a carbon source that is combined with a metal catalyst made of powder forge nickel and powder forge cobalt. Previously used targets involved pressing and binding targets with Dylon carbon cement. These particles had an approximate 200-micron particle size, which led to large regions of uncatalyzed target. The new metal powders are small (~0.5 microns), highly dispersible, and two orders of magnitude less expensive. The powders are mixed in appropriate quantities and subjected to low-energy ball-milling to
ensure mingling. Targets undergo additional preparatory steps, including high pressure processing. The targets are then subjected to a series of ultrafast infrared laser light pulses at a high repetition rate to vaporize the layers of the spinning targets and create a plume of nanotubes.

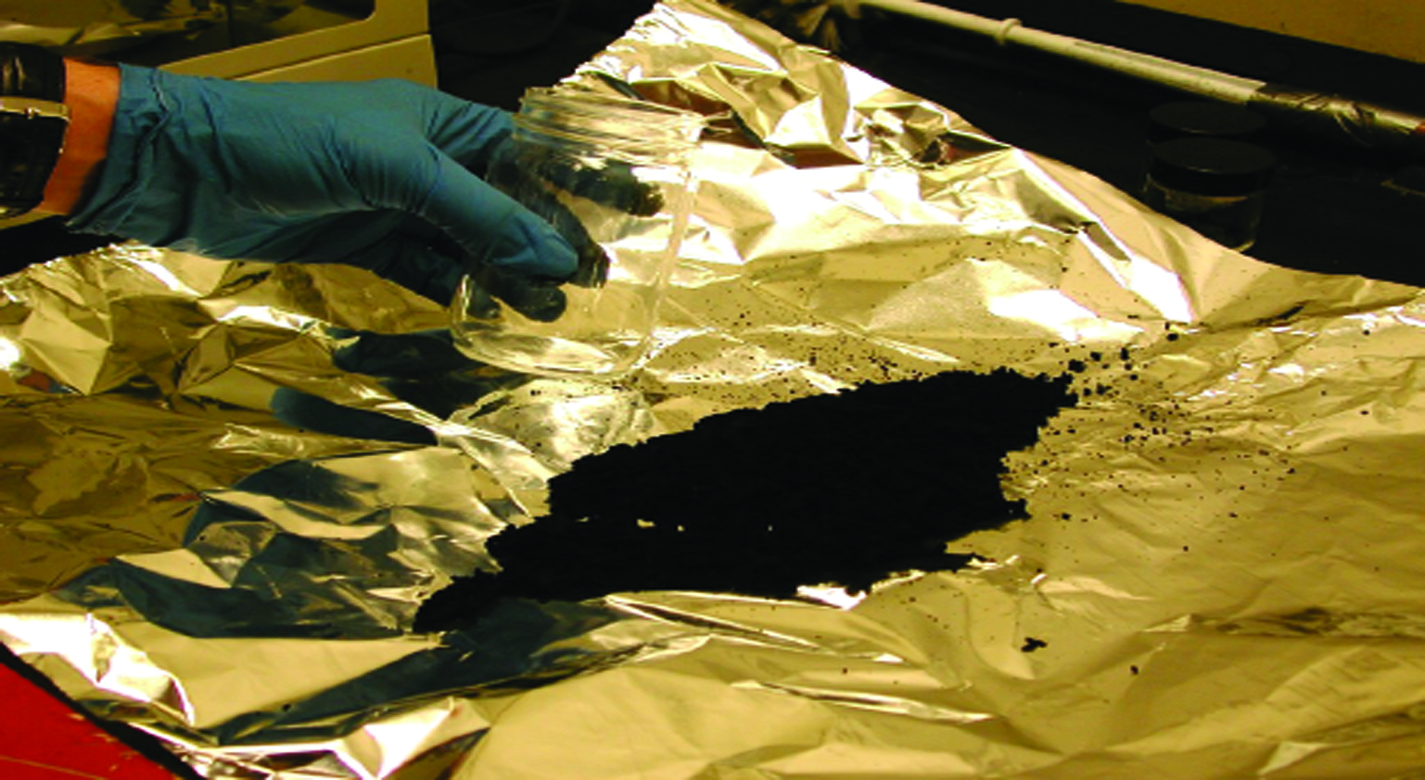
Benefits
- Uses inexpensive material to make carbon nanotubes
- Facilitates high-yield nanotube production
- Very small and dispersible targets enable more complete catalysis
- Targets are significantly less expensive than those currently used
- Nanotubes are straight, homogenous, and defect-free
- Targets produce high quality and research grade carbon nanotubes
Applications
- These targets will facilitate the production of high quality single-walled carbon nanotubes in large quantities synthesized via the FEL technique. Carbon nanotubes of this nature and size have been difficult to make in high yield, limiting their commercial viability. This advancement opens the door to potential industry applications that have so far not been conceived. An initial application would be in fiber-reinforced composites used in aerospace.
Similar Results

Surface Densification Of Phenolic Impregnated Carbon Ablator (PICA)
The graded Thermal Protection System (TPS) offers a lower density than comparable state-of-the-art TPS systems operating at similar maximum heating conditions. This approach is straight forward in terms of processing and surface-treatment application and can be applied to machine PICA materials without having an effect on the final tolerance. The process results in increased usability and handling since standard uncoated PICA is relatively weak. Surface-densified PICA provides an approach for improvements in the robustness for the baseline CEV heat shield. A graded approach eliminates the need for joints and/or bonding agents between material plies. PICA surface densification offers robust mechanical protection against transit damage, handling damage, and in-flight object damage.

Low-Temperature Oxidation/
Reduction Catalysts
The low-temperature oxidation catalyst technology employs a novel catalyst formulation, termed platinized tin oxide (Pt/SnOx). The catalysts can be used on silica gel and cordierite catalyst supports, and the latest developments provide sprayable formulations for use on a range of support types and shapes. Originally developed for removal of CO, the catalyst has also proven effective for removal of formaldehyde and other lightweight hydrocarbons.
NASA researchers have also extended the capability to include reduction of NOx as well as developed advanced chemistries that stabilized the catalyst for automotive catalytic converters via the engineered addition of other functional components. These catalyst formulations operate at elevated temperatures and have performed above the EPA exhaust standards for well beyond 25,000 miles. In addition, the catalyst can be used in diesel engines because of its ability to operate over an increased temperature range.
For use as a gas sensor, the technology takes advantage of the exothermic nature of the catalytic reaction to detect formaldehyde, CO, or hydrocarbons, with the heat being produced proportional to the amount of analyte present.

Multi-Layer Nuclear Thermionic Avalanche Cell
The Multi-Layer NTAC is comprised of a gamma-ray source and various layers of emitters, collectors, and insulators. Ideal emitter materials include elements with high atomic numbers, while ideal collector and insulator materials include elements with low atomic numbers. A high-energy gamma-ray (tens of keV to MeV) is used to liberate a large number of intra-band, inner-shell electrons from atoms within the emitter material for power generation through the primary interactions of photoelectric, Compton scattering, photonuclear, and electron/positron pair production processes. Secondary and tertiary electrons are liberated in the avalanche process as well. If a power conversion process effectively utilizes all liberated electrons in an avalanche mode through a power conversion circuit, the power output is drastically increased. Because power conversion is determined by the absorption rate of high energy photons, increasing power output requires either thicker collector material or a sufficient number of layer structures to capture the high energy photons, leaving no liberated electrons escaping (i.e., minimizing the leak of radioactive rays). The selection of materials, the thicknesses of the emitter, collector, and insulator, as well as the number of NTAC layers required are all determined by the energy of photon source. The thermal energy from radioactive decay can also be converted to electricity using a thermoelectric device to further increase power output. The Multi-Layer NTAC technology can be manufactured using existing semiconductor fabrication technology and can be tailored for small-to-large scale power needs, including kilowatt and megawatt applications.

Holey Carbon Allotropes
This invention is for scalable methods that allows preparation of bulk quantities of holey nanocarbons with holes ranging from a few to over 100 nm in diameter. The first method uses metal particles as a catalyst (silver, copper, e.g.) and offers a wider range of hole diameter. The second method is free of catalysts altogether and offers more rapid processing in a single step with minimal product work-up requirements and does not require solvents, catalysts, flammable gases, additional chemical agents, or electrolysis. The process requires only commercially available materials and standard laboratory equipment; and, it is scalable. Properties that can be controlled include: surface area, pore volume, mechanical properties, electrical conductivity, and thermal conductivity.

Dispersion of Carbon Nanotubes in Polymers
The technology portfolio spans several methods for dispersion and processing of CNTs in polymer resins and composites. CNT/resin systems with high dispersion and long-term stability are provided by three general approaches. One method relies on mechanical dispersion by sonication simultaneous with partial polymerization to increase the resin viscosity to maintain dispersion and enable further polymer processing of the CNT blend into films and other articles. Another approach relies on what is termed donor acceptor bonding, which essentially is a dipole bond created on the CNT/resin interface to maintain dispersion and stability of the CNT/resin blend. This dispersion method also provides advantages in mechanical properties of processed composites due to the interface characteristics. A range of polymer types can be used, including polymethyl methacrylate, polyimide, polyethylene, and others.
An additional dry blending approach provides advantages for a variety of
thermoplastic and thermoset systems. Use of ball mill mixing achieves effective
blending and dispersion of the CNT, even at high loadings. Further processing steps
using injection molding or similar melt processing methods have yielded CNT/
polymer composites with a range of useful electronic, optical, and mechanical
properties.