Surface Densification Of Phenolic Impregnated Carbon Ablator (PICA)
materials and coatings
Surface Densification Of Phenolic Impregnated Carbon Ablator (PICA) (TOP2-183)
Modification of Surface Density of a Porous Medium
Overview
This technology from NASA allows a graded-surface densification of Phenolic Impregnated Carbon Ablator (PICA). PICA was developed at NASA Ames Research Center in the 1980s for the forebody heatshield of the Stardust Return Capsule. With a low density (~0.27g/cm), coupled with efficient ablative capability at high heat fluxes, PICA became an enabling technology for the Stardust mission. At the time of the mission, PICA was a developmental material, with no previous flight heritage. For missions with high heat flux, a lower overall mass can be obtained if the Thermal Protection System (TPS) composition changes from a carbon-based ablator at the heated surface to an insulator near the inner surface. This new process delivers a graded surface-densification application to PICA allowing for optimized performance and TPS weight reduction. The desired surface densification is adaptable in terms of density of the applied surface treatment and depth of the surface treatment.
The Technology
The graded Thermal Protection System (TPS) offers a lower density than comparable state-of-the-art TPS systems operating at similar maximum heating conditions. This approach is straight forward in terms of processing and surface-treatment application and can be applied to machine PICA materials without having an effect on the final tolerance. The process results in increased usability and handling since standard uncoated PICA is relatively weak. Surface-densified PICA provides an approach for improvements in the robustness for the baseline CEV heat shield. A graded approach eliminates the need for joints and/or bonding agents between material plies. PICA surface densification offers robust mechanical protection against transit damage, handling damage, and in-flight object damage.

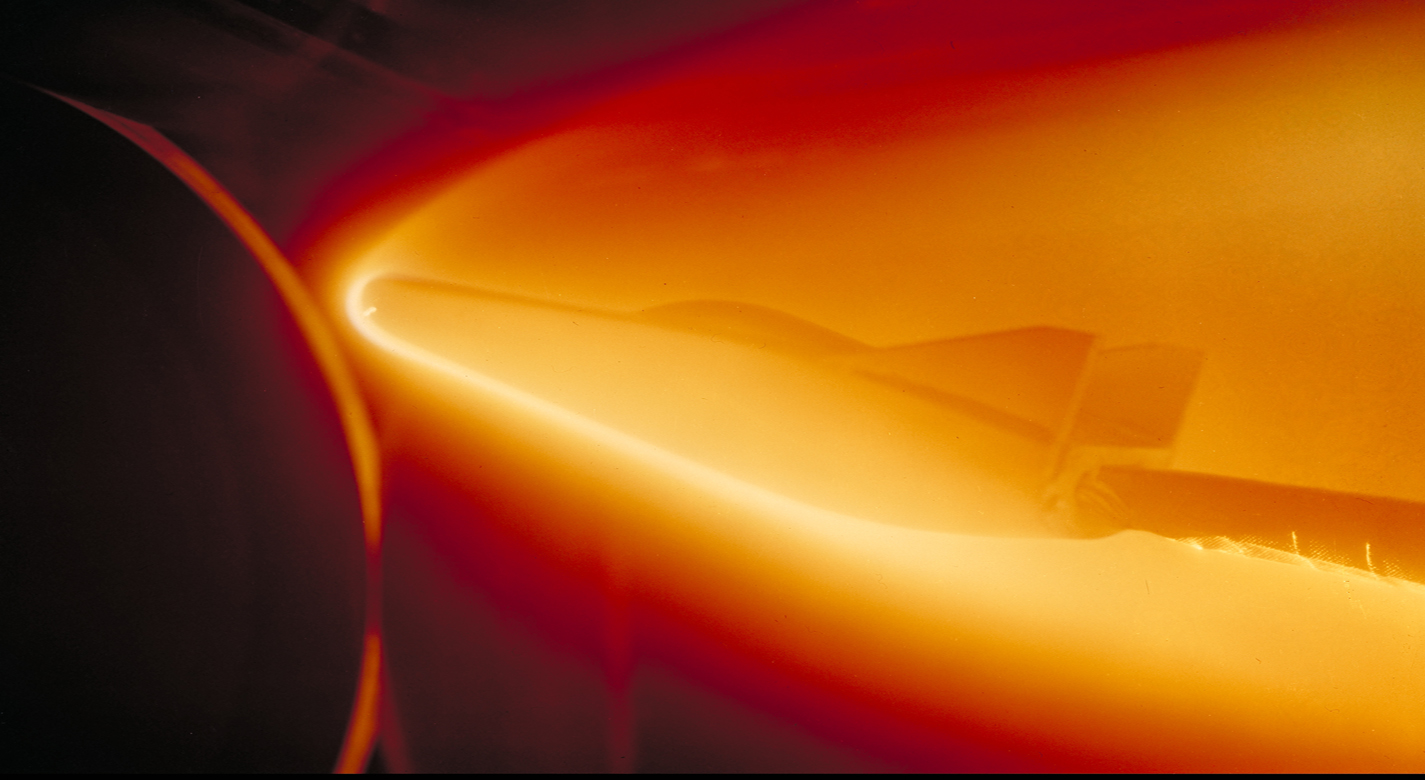
Benefits
- Much lower density than other carbon/phenolic systems
- Efficient ablative capability at high heat fluxes
- Design optimization using composite response surfaces
- Improved robustness
- Applied without having an effect on the final tolerance
- Optimization of surface densification as needed
- Allows for monolithic and multi-tile thermal protection system designs for planetary entry or re-entry heat shield applications
Applications
- Space exploration
- Systems engineering
- Thermal Protection Systems
- Materials engineering
- Mechanical engineering
Similar Results

New Resin Systems for Thermal Protection Materials
This method produces a low density ablator similar to Phenolic Impregnated Carbon Ablator (PICA) using a cyanate ester and phthalonitrile resin system, rather than the heritage phenolic resin. Cyanate ester resin systems can be cured in a carbon matrix and generate high surface area structure within the carbon fibers. This helps to reduce the thermal conductivity of the material which is one of the key requirements of thermal protection system (TPS) materials. The material has densities ranging from 0.2 to .35 grams per cubic centimeter. NASA has successfully processed the cyanate ester and phthalonitrile resins with a morphology similar to that of the phenolic phase in PICA, but with more advanced properties such as high char stability, high char yield, and high thermal stability. This new generation of TPS materials has the same microstructure as heritage PICA, but improved characteristics of PICA such as increased char yield, increased char stability, increased thermal stability and increased glass transition temperature.

In-Situ Resource Utilization (ISRU): Methylotrophic Microorganisms Expressing Soluble Methane Monooxygenase Proteins
Microorganisms are unique from the standpoint that they can be employed as self-replicating bio-factories to produce both native and engineered mission relevant bio-products. Methane (CH4) usage in In-Space Manufacturing (ISM) platforms has been discussed previously for human exploration and has been proposed to be used in physicochemical systems as a propulsion fuel, supply gas, and in fuel cells. Carbon Dioxide (CO2) is abundant on Mars and manned spacecraft. On the International Space Station (ISS), NASA reacts excess CO2 with Hydrogen (H2) to generate CH4 and Water (H2O) using the Sabatier System (Figure 1). The resulting water is recovered in the ISS, but the methane is vented to space. Recapturing this methane and using it for microbial manufacturing could provide a unique approach in development of in-space bio-manufacturing. Thus, there is a capability need for systems that convert methane into valuable materials. Methane (CH4) is a potential carbon substrate for methanotrophic microorganisms which are able to metabolize CH4 into biomass. The innovative technology from NASA Ames Research Center ports Soluble Methane Monooxygenase (sMMO) to Pichia, that is, it moves the methane metabolism into a robust microbial factory (Pichia pastoris) (Figure 2). The yeast Pichia pastoris is a refined microbial factory that is used widely by industry because it efficiently secretes products. Pichia could produce a variety of useful products in space. Pichia does not consume methane but robustly consumes methanol, which is one enzymatic step removed from methane. This novel innovation engineers Pichia to consume methane thereby creating a powerful methane-consuming microbial factory and utilizing methane in a robust and flexible synthetic biology platform.

A New Family of Low-Density, Flexible Ablators
The invention provides a family of low density, flexible ablators comprising of a flexible fibrous substrate and a polymer resin. The flexible ablators can withstand a wide range of heating rates (40-540 Watts/cm2) with the upper limit of survivable heat flux being comparable to the survivable heat flux for rigid ablators, such as PICA and Avcoat. The amount and composition of polymer resin can be readily tailored to specific mission requirements. The material can be manufactured via a monolithic approach using versatile manufacturing methods to produce large area heat shields, which provides a material with fewer seams or gaps. The goals of the work are primarily twofold: (i) to develop flexible, ablative Thermal Protection System (TPS) material on a large, blunt shape body which provides aerodynamic drag during hypervelocity atmospheric entry or re-entry, without perishing from heating by the bow shock wave that envelopes the body; and (ii) to provide a relatively inexpensive TPS material that can be bonded to a substrate, that is unaffected by deflections, by differences in thermal expansion or by contraction of a TPS shield, and that is suitable for windward and leeward surfaces of conventional robotic and human entry vehicles that would otherwise employ a rigid TPS shield. This technology produces large areas of heat shields that can be relatively easily attached on the exterior of spacecraft.

Low-Temperature Oxidation/
Reduction Catalysts
The low-temperature oxidation catalyst technology employs a novel catalyst formulation, termed platinized tin oxide (Pt/SnOx). The catalysts can be used on silica gel and cordierite catalyst supports, and the latest developments provide sprayable formulations for use on a range of support types and shapes. Originally developed for removal of CO, the catalyst has also proven effective for removal of formaldehyde and other lightweight hydrocarbons.
NASA researchers have also extended the capability to include reduction of NOx as well as developed advanced chemistries that stabilized the catalyst for automotive catalytic converters via the engineered addition of other functional components. These catalyst formulations operate at elevated temperatures and have performed above the EPA exhaust standards for well beyond 25,000 miles. In addition, the catalyst can be used in diesel engines because of its ability to operate over an increased temperature range.
For use as a gas sensor, the technology takes advantage of the exothermic nature of the catalytic reaction to detect formaldehyde, CO, or hydrocarbons, with the heat being produced proportional to the amount of analyte present.

Advanced Isothermally Produced Next-Gen Composites
Next generation aircraft are anticipated to be largely made with composite components, requiring significant increases in manufacturing rates of composites to meet the demand for a new fleet of aircraft. The higher rate manufacturing will require multiple advances, including rapid curing and lower processing temperatures. These requirements can be enabled by new processing methods such as isothermal rapidly cured composite parts.
NASA has developed materials and methods that meet those stringent requirements for high-rate manufacturing. The innovators have demonstrated at least two families of new resin formulations that meet the expected high-rate manufacturing needs. These new formulations have been engineered to be infused and cured at the same (i.e., isothermal) temperature, below that of commercially available materials. The materials can then be removed from the mold while still hot without distorting the shape, thereby reducing the processing times by eliminating the need for cooling to occur in the mold. After a post-cure process - which takes 4 hours or less and can be performed in batches - the mechanical properties of NASA's next-gen composites.
The related patent is now available to license. Please note that NASA does not manufacturer products itself for commercial sale.