The Teletenna - A Hybrid Telescope Antenna System
communications
The Teletenna - A Hybrid Telescope Antenna System (LEW-TOPS-118)
Enables deep space missions by combining radio frequency and optical communications
Overview
Innovators at NASA's Glenn Research Center have developed a hybrid telescope antenna system - Teletenna - to deliver high data-rate communication over great distances. Teletenna has the potential to benefit deep space missions and communications on Earth. By combining two very different communications systems - optics and radio frequency (RF) - Teletenna capitalizes on the benefits of each system while overcoming conflicting engineering requirements. Teletenna is a breakthrough innovation, particularly in the field of Deep Space Optical Communications (DSOC), in which it could deliver high-definition imagery, live video feed, and real time data-transmission 10 to 100 times faster than current state-of-the-art technology. Teletenna supports beaconless pointing, remains nearly transparent to RF, and achieves an unprecedented level of data richness and bandwidth. This exceptionally lightweight and precise instrument stands ready to revolutionize deep space exploration, satellite communications, telecommunications, and more.
The Technology
Initially developed for missions to Mars, Teletenna integrates RF and optical communication technologies to transmit data from deep space to Earth at extremely high speeds. The system combines a co-boresighted telescope and a Ka-band RF antenna to minimize system mass and enhance performance. Designed with an optimal focal length-to-diameter ratio, the apparatus features a classical Cassegrain geometry, including a sub-reflector in front of the RF feed which acts as a mirror for the optical signal while being transparent to the RF signal. The apparatus also mechanically and thermally isolates the RF reflector system from the optics to offer maximum stability.
Teletenna was created to overcome two significant challenges to DSOC: 1) laser inefficiency due to poor alignment during spacecraft disturbances and 2) performance degradation due to lack of rigidity in vibrational environments (such as space). The first challenge is addressed by the telescope portion of this technology, which facilitates the acquisition and maintenance of the link with ease - even in less than ideal conditions. The second challenge is addressed by rigidly fixing the RF reflector to the spacecraft body and attaching the optical section to a vibration isolation platform. The result is a device that can point to within 0.5 degrees of the sun (traditional optical systems are limited to 3 degrees), allowing for approximately 20 extra days of contact time between Earth and Mars. By combining RF and optical communications, this breakthrough innovation has the power to transform communications as we know it.
Glenn welcomes co-development opportunities.
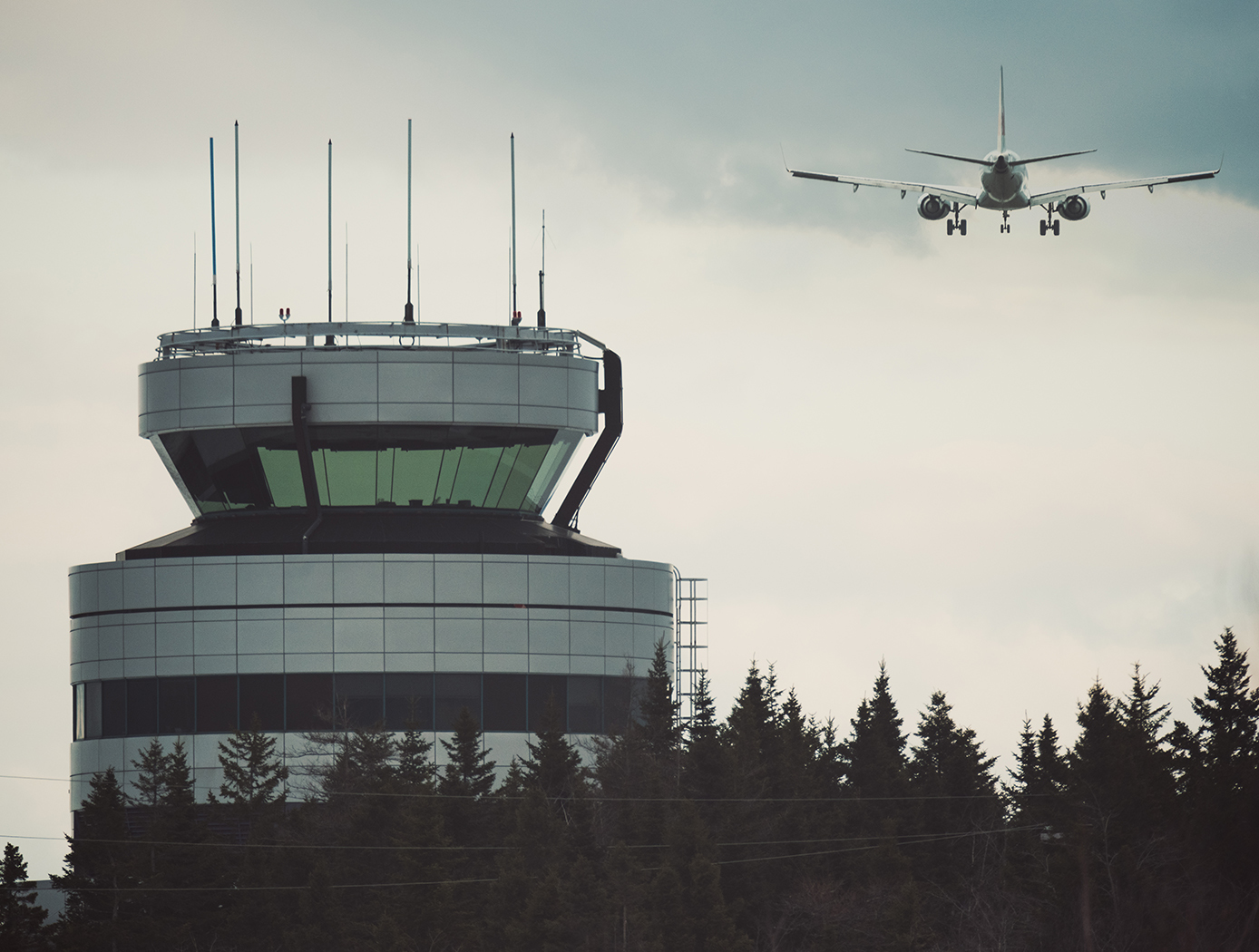
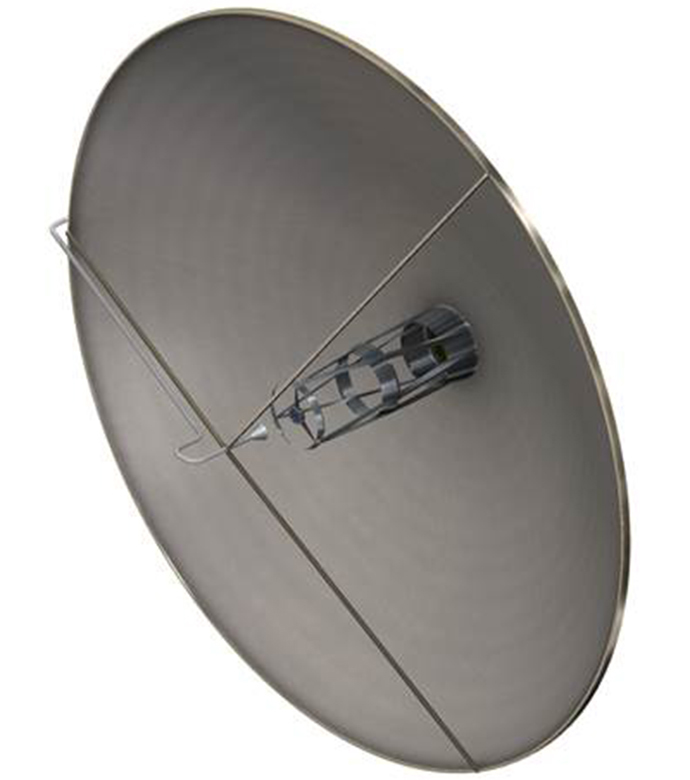
Benefits
- Reliable: Combines the benefits of high data-rate optics with the reliability of a RF system
- Low Mass: Achieves the functionality of both a telescope and an antenna with minimal system mass
- High Data Rates: Can return data at rates approaching 300 Mbps optically and 100 Mbps RF at Mars' closest approach
- Robust: Provides optical alignment and stabilization in high vibration environments and is tolerant to radiation
- Precise: Combines Ka-band RF with 1550 nanometer optical capability
Applications
- Aerospace
- Secure communications for aircraft
- Satellites
Technology Details
communications
LEW-TOPS-118
LEW-19485-1
LEW-19647-1
LEW-19666-1
Similar Results

Dual S-band and Ka-band High Gain Antenna
The circularly polarized antenna features an integrated prime-fed S-band and Cassegrain-based Ka-band reflector system. The Cassegrain primary and secondary reflectors are specially shaped for optimal Ka-band gain, while a frequency selective surface on the secondary reflector provides reflectivity at Ka-band, and acts as a transparent dielectric radome for the S-band feed antenna. Design innovations include an improved S-band feed antenna and cross-polarization compensation, improved Ka-band horn, and special
shaping of the secondary reflector for ease of fabrication.
The technology improves upon prior S-band feed antenna designs to provide mechanical robustness and low cross-polarization over a wide field of view. It also improves the front-to-back ratio, providing much higher signal radiated forward, over an increased bandwidth as well. The Ka-band feed horn is based on a prior NASA innovation, the standard Potter horn, but in this innovation has significantly lower sidelobe level performance. This is achieved by using a modified smooth s-curved interior horn profile rather the the typical conical/cylindrical form typical of the standard Potter horn design. The smooth interior wall is also easier to fabricate than alternative corrugated wall designs. Additionally, a cross-polarization cancellation cup is integrated with the Ka-band horn geometry, with the cup being placed around the neck of the horn in the form of a collar, allowing the two to be fabricated together.

High-Speed, Low-Cost Telemetry Access from Space
NASA's SDR uses Field-Programmable Gate Array (FPGA) technology to enable flexible performance on orbit. A first-generation FM-modulated transceiver is capable of operating at up to 1 Mbps downlink and 50 kbps uplink, full duplex. An FPGA performs Reed-Solomon (255,223) encoding, decoding, and bit synchronization, providing Consultative Committee for Space Data Systems (CCSDS) and Near Earth Network (NEN) telemetry protocol compatibility. The transceiver accepts data from the onboard flight computer via a source synchronous RS422 interface.
NASA's second-generation full duplex SDR, known as PULSAR (programmable ultra-lightweight system-adaptable radio, Figures 1 and 2 below) incorporates command receiver and telemetry transmitters, as well as updated processing and power capabilities. An S-band command receiver offers a max uplink data rate of 300 Kbps and built-in QPSK demodulation. X- and S-Band telemetry transmitters offer a max downlink data rate of 150 Mbps and flexible forward-error correction (FEC) using Reed-Solomon encoding (LDPC rate 7/8 and 1/2 convolution in development), and it uses QPSK modulation. The use of FEC adds an order of magnitude increase in telemetry throughput due to an improved coding gain. An onboard FPGA uses high-speed logic for uplink/downlink and encoding/decoding processes. Balloon flight testing has been conducted and is ongoing for PULSAR.

Lightweight, Self-Deployable Helical Antenna
NASA's newly developed antenna is lightweight (at or below 2 grams), low volume (at or below 1.2 cm3), and low stowage thickness (approx. 0.7 mm), all while delivering high performance (at or above 10 dBi gain). The antenna includes a novel design-material combination in a helical coil conformation. The design allows the antenna to compress for stowage (e.g., satellite launch), then self-deploy at the desired time in orbit.
NASA's lightweight, self-deployable helical antenna can be integrated into a thin-film solar array (or other large deployable structures). Integrating antenna elements into deployable structures such as power generation arrays allows spacecraft designers to maximize the inherently limited resources (e.g., mass, volume, surface area) available in a small spacecraft. When used as a standalone (i.e., single antenna) setup, the the invention offers moderate advantages in terms of stowage thickness, volume, and mass. However, in applications that require antenna arrays, these advantages become multiplicative, resulting in the system offering the same or higher data rate performance while possessing a significantly reduced form factor.
Prototypes of NASA's self-deployable, helical antenna have been fabricated in S-band, X-band, and Ka-band, all of which exhibited high performance. The antenna may find application in SmallSat communications (in deep space and LEO), as well as cases where low mass and stowage volume are valued and high antenna gain is required.

Multimode Directional Coupler
Glenn's researchers originally created the MDC to improve the beacon sources for atmospheric propagation studies. These studies are typically conducted to test atmospheric conditions to determine the signal strength needed for satellite communications. A low-power transmitter (e.g., a beacon source) is attached to the satellite, and transmits a continuous waveform (CW) signal to a receiving station on Earth. However, when a separate frequency is desired, building a new beacon source for the transmitter on the satellite - especially one that will operate at higher frequencies - presents numerous challenges. For one, a single-frequency beacon source requires a temperature-stabilized oscillator for frequency generation separate from that provided by the spacecraft receiver.
To solve such problems, Glenn's innovators fabricated the MDC from two sections of waveguide: a primary waveguide for the fundamental frequency (Ku-band), and a secondary waveguide for the harmonics (Ka-band). These sections are joined together so that precision-machined slots in the second waveguide selectively couple the harmonics, for amplification and transmission. The harmonics can then be used as an additional beacon source with very small power losses to the fundamental signal. Once the separation takes place, the second or higher harmonic can be amplified and transmitted to a station on Earth. The efficiency and performance of the MDC can be optimized through appropriate computer modeling software and currently available high-precision fabrication techniques. Without the complexity and expense involved in building separate traveling wave tube amplifiers to generate additional frequencies, Glenn's MDC enables satellites to produce multiple signals that can be received by multiple stations - a significant leap forward in satellite productivity.

Tunable Multi-Tone, Multi-Band, High-Frequency Synthesizer
Glenn's revolutionary new multi-tone, high-frequency synthesizer can enable a major upgrade in the design of high data rate, wide-band satellite communications links, in addition to the study of atmospheric effects. Conventional single-frequency beacon transmitters have a major limitation: they must assume that atmospheric attenuation and group delay effects are constant at all frequencies across the band of interest. Glenn's synthesizer overcomes this limitation by enabling measurements to be made at multiple frequencies across the entire multi-GHz wide frequency, providing much more accurate and actionable readings.
This novel synthesizer consists of a solid-state frequency comb or harmonic generator that uses step-recovery semiconductor diodes to generate a broad range of evenly spaced harmonic frequencies, which are coherent and tunable over a wide frequency range. These harmonics are then filtered by a tunable bandpass filter and amplified to the necessary power level by a tunable millimeter-wave power amplifier. Next, the amplified signals are transmitted as beacon signals from a satellite to a ground receiving station. By measuring the relative signal strength and phase at ground sites the atmospheric induced effects can be determined, enabling scientists to gather essential climate data on hurricanes and climate change. In addition, the synthesizer can serve as a wideband source in place of a satellite transponder, making it easier to downlink high volumes of collected data to the scientific community. Glenn's synthesizer enables a beacon transmitter that, from the economical CubeSat platform, offers simultaneous, fast, and more accurate wideband transmission from space through the Earth's atmosphere than has ever been possible before.