Conductive High-Toughness Oxides
materials and coatings
Conductive High-Toughness Oxides (LEW-TOPS-132)
Metastable phases provide unique coatings with durable, conductive, and insulating properties
Overview
Innovators at NASA's Glenn Research Center have developed a novel method for making oxide coatings that have excellent mechanical strength and electronic conductivity at room temperature. Using the Plasma Spray-Physical Vapor Deposition (PS-PVD) processing methodology, Glenn researchers have been able to produce a coating containing a metastable phase that no other method can achieve. Upon exposing the material to moderate temperatures (greater than 300°C) in the presence of oxygen, the metastable phase is further oxidized, and the material becomes insulating while retaining its high toughness and microstructure. With this innovation, a coating can be created from any metal oxide, including rare earth oxides, zirconium oxide, hafnium oxide, titanium oxide, or any combination of two or more oxides. Glenn's innovation has great potential for industries that require increasingly tough ceramic coatings, such as electronics, chemical and petroleum refineries, the aerospace industry, and automotive and aeronautics manufacturing.
The Technology
Oxide coatings have been used in thermal and environmental barrier layers for coatings for hot section turbine applications, among other uses. With the PS-PVD method, Glenn researchers observed the formation of a minority phase of a metastable oxide (zirconium oxide) that is usually found only in a vapor state. They found that the high temperatures and fast deposition process of the PS-PVD system incorporated nonequilibrium phases in the coating and retained them at room temperature as well as at high temperature in the absence of oxygen. The material is vaporized and condensed on the surface via a rapid quenching, essentially "trapping" this phase in the deposited coating. The coating microstructure and composition can also be manipulated by changing the processing parameters, allowing the thickness of the coating to be tailored to a given application. Since this metastable phase is conductive, this coating can be used as (for example) an extremely sensitive (thermal or temperature) sensor. It also has very good durability and erosion resistance, making it useful as a protective and conductive coating for electronics and microelectronics. This is an early-stage technology requiring additional development, and Glenn welcomes co-development opportunities.
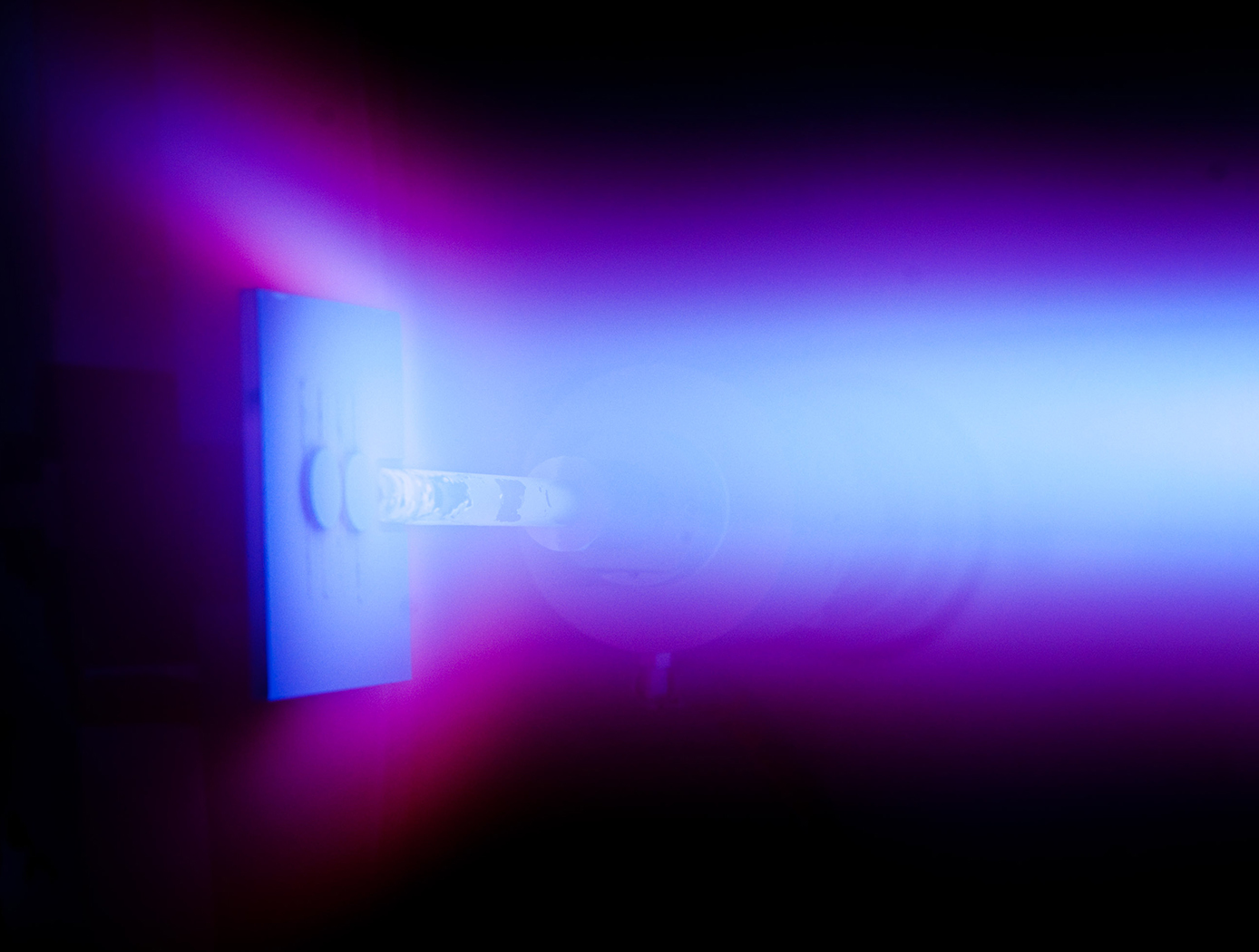
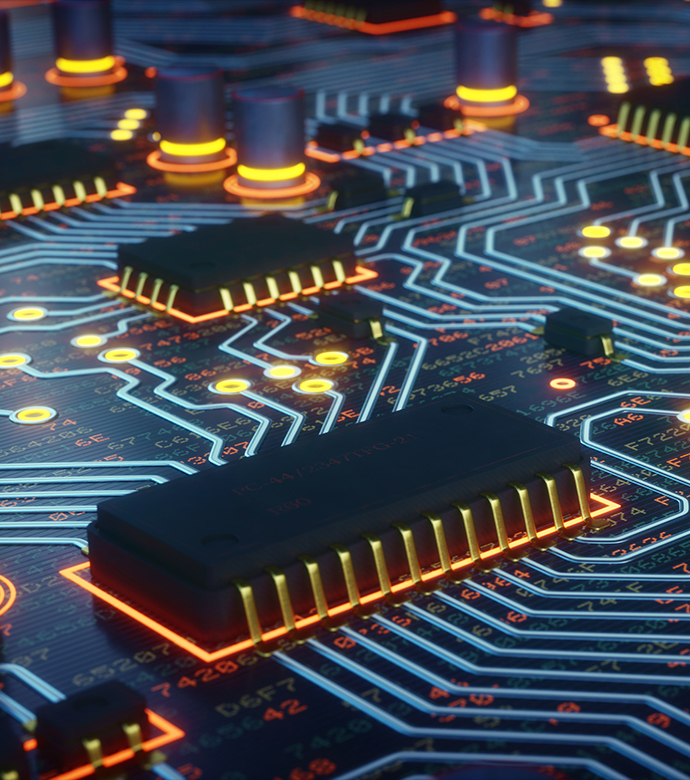
Benefits
- Durable: Has very good toughness and erosion resistance
- Efficient: Combines low thermal conductivity with electrical conductivity
- Flexible: Can change coating composition and microstructure by changing the processing parameters and can coat complex shapes with limited manipulation
- Simple: Can be deposited in a single step
- Uniform and Dense: Suitable for porous and non-porous metals and ceramics, turbine engine components, and SiC/SiC semiconductors substrates/devices
Applications
- Aerospace
- Automotive
- Chemical manufacturing
- Coatings (e.g., heat and environmental protection)
- Electronics (e.g., thermal cut-off, thermistors)
- Filters (e.g., gas separation membranes)
- Insulation
- Oil and gas
- Power (e.g., ionic conduction membranes)
- Semiconductors
Similar Results

Fabricating printable electronics and biosensor chips
The plasma system consists of a glass tube with a diameter of 0.5 mm or larger, if desired. The electrodes are separated by 10 mm. Helium, argon or cold dry air can be used as a plasma gas source. An applied high voltage between the electrodes causes the gas to breakdown within the central core of the glass capillary generating atmospheric plasma. Nanostructures colloids/organic/inorganic precursors are placed in a glass container with an inlet and outlet for carrier gas and are seated on an ultrasonic nebuliser. The aerosol is then carried into the plasma stream by the carrier gas and is deposited.
The atmospheric plasma deposition system can be modified for depositing multiple materials, either simultaneously or sequentially, and for high-throughput processing by having multiple jets. Each capillary can either be connected to the container containing a single precursor material or to different containers containing different precursor materials to facilitate multiple depositions. The multi-jet plasma system can be automated and controlled individually to precisely control surface characteristics. This technique is independent of the chosen substrate, and has proven to work for many substrates, including paper, plastic, semiconductors and metals.

Oil-Free Lubricants
In applying PS400 using the plasma spray-coating process, a 0.010 inch layer is deposited onto a metal surface. This composite coating often includes a metallic-based binder, a metal-bonded hardener, a high-temperature lubricant, and/or a low- temperature lubricant. PS400's improved metallic-based binder alloy greatly increases the structural strength and durability of the composite with respect to the operating temperature and the bearing load, and provides superior dimensional stability. PS400's metal-bonded oxide hardening agent provides additional hardness, wear resistance, and thermal stability, while also exhibiting a low coefficient of friction when used in sliding contacts. It is also significantly less expensive in terms of both acquisition and grinding processes.
Depending on the desired environment, high- and low-temperature lubricants may be added to the composite coating. The preferred high-temperature lubricant is a metal fluoride and the optional optional low-temperature lubricant is composed of metals, such as silver or copper, that are soft enough to provide lubrication at low temperature while maintaining oxidation resistance with a sufficiently high melting point. These qualities permit the materials to be used over a broad temperature range. Once the spray coating has been applied, the metal surface is ground and polished to produce a smooth, self-lubricating surface before use. Unlike some coatings that must be diamond-ground, PS400 is readily ground with a substantially less expensive abrasive, such as silicon carbide. This grinding process generally yields a coating thickness of 200 to 400 micrometers.
In instances when a coating is not convenient or possible, powder metallurgy techniques using PM400 can be used to make freestanding self-lubricating components such as bushings and wear plates.

Cryogenic Selective Surfaces
These materials, which are composed of highly optically transmissive materials, are engineered to provide near-perfect reflection of the full solar spectrum in space. The materials are finely divided such that they scatter and reflect the incoming radiation from the UV down into the mid-IR and are also coated in some fashion with silver to extend the reflectance down into the far IR region of the solar spectrum. With this near-perfect reflectance of the complete solar spectrum, the scientists envision use of these materials for maintaining cryogenic temperatures for extended periods of time in space. The materials have also been developed into highly flexible, moisture resistant selective surface paint.The use and storage of cryogenics fluids is critical to many space operations, and while there are thermal control coatings in use today for spacecraft, none can provide this near-perfect reflection required for long-term maintenance of cryogenic temperatures.

High Atomic Number Coatings for Fabrics
High atomic number materials, such as tantalum, do not bond well to oxygen- and hydroxyl-rich surfaces, such as glass fibers. These metals often form surface oxides when layered on glass fabric, resulting in flaking of the high atomic number material off the fabric during cutting, folding, and/or handling.
To improve coating durability, this invention applies a lower atomic number metal as a tie down layer first before applying the high atomic number metal layer. The tie down layer reduces oxide formation between the substrate and the high atomic number material, promoting adhesion. Titanium has shown strong adhesion with different metals and is effective at reducing oxide formation when diffusion bonded to itself or other materials. It has been shown to be effective at improving durability when thermally sprayed onto a glass fiber fabric as a tie down layer for a subsequent tantalum layer (also applied via RF plasma spray). The titanium layer is only approximately 1 mil thick but results in strong adhesion of the tantalum layer by inter-metallic or diffusion bonding. A thermal spray process may be used, as well.
This innovation enables the delivery of high atomic metal coating on glass fiber fabrics and other polymeric substrates that are lower cost, lighter weight, and durable to form a flexible cloth material with Z-graded radiation shielding. Coated samples have been produced and the technology is currently at a technology readiness level (TRL) of 4 (prototype).

Environmental Barrier Coatings for Ceramic Matrix Composites
CMCs are a game-changer for a number of applications because of their lighter weight, higher temperature capability, and resistance to oxidation. It has been estimated that aircraft designs relying on CMCs can decrease fuel consumption by 10% by 2020. EBCs are used to protect CMCs from water vapor and other corrosive gases inside engines and other extreme environments. The current state of the art for EBCS features a silicon bond coat that is not viable beyond its melting point of 1482°C. By contrast, Glenn's EBCs have demonstrated a steam oxidation life of at least 500 hours at 1482°C, making them ideal durable coatings for next-generation CMCs. These EBCs are slurries, with either a mullite-based bond coat or a rare earth disilicate-based bond coat comprising at least three and two layers, respectively. Mullite is often used as a refractory material for furnaces, reactors, etc. because of its high melting point (1840°C). Rare earth disilicates also have high melting points (~1800°C). These bond coats can be fabricated by preparing a mixture of a coating material, a primary sintering aid, at least one secondary sintering aid, and a solvent. The mixture is then processed (e.g., in a milling media) to form a slurry that can be deposited to a CMC substrate. The sintering aids have two primary functions: 1) densifying deposited slurry by generating liquid phases via reactions with the coating material and other sintering aids, so that the liquid fills gaps between particles of coated material; 2) enhancing bonding and performance of the coating by generating reaction products that enhance those qualities. One great advantage of this EBC is that it can be fabricated via various low-cost methods - including dipping, spinning, spin-dipping, painting, and spraying - in addition to plasma-spraying. Glenn's innovation rises to meet the need for a new class of EBCs that can keep up with CMCs' increasing ability to withstand higher temperatures and stresses than ever before.