Environmental Barrier Coatings for Ceramic Matrix Composites
materials and coatings
Environmental Barrier Coatings for Ceramic Matrix Composites (LEW-TOPS-136)
Protect high-performance components in extreme environments
Overview
Innovators at NASA's Glenn Research Center have developed two durable environmental barrier coatings (EBCs) for use with ceramic matrix composite (CMC) components. CMCs are lightweight composites used to reduce fuel consumption in advanced gas turbines and other high-temperature, high-stress environments (up to 1482°C). Glenn's breakthrough EBCs are the only coatings that can withstand such temperatures, protecting CMCs from increased wear and corrosion, oxidation, and water vapor recession in extreme environments. In addition, these EBCs can be fabricated with simpler and lower-cost methods than conventional coating processes such as plasma spraying. Glenn's EBCs add reliability and lifespan to CMCs' advantages of lighter weight, higher temperature capability, and greater oxidation resistance. This innovation is poised to facilitate the upcoming CMC revolution, from hot components in next-generation, fuel-efficient jet engines to furnaces to nuclear reactors.
The Technology
CMCs are a game-changer for a number of applications because of their lighter weight, higher temperature capability, and resistance to oxidation. It has been estimated that aircraft designs relying on CMCs can decrease fuel consumption by 10% by 2020. EBCs are used to protect CMCs from water vapor and other corrosive gases inside engines and other extreme environments. The current state of the art for EBCS features a silicon bond coat that is not viable beyond its melting point of 1482°C. By contrast, Glenn's EBCs have demonstrated a steam oxidation life of at least 500 hours at 1482°C, making them ideal durable coatings for next-generation CMCs. These EBCs are slurries, with either a mullite-based bond coat or a rare earth disilicate-based bond coat comprising at least three and two layers, respectively. Mullite is often used as a refractory material for furnaces, reactors, etc. because of its high melting point (1840°C). Rare earth disilicates also have high melting points (~1800°C). These bond coats can be fabricated by preparing a mixture of a coating material, a primary sintering aid, at least one secondary sintering aid, and a solvent. The mixture is then processed (e.g., in a milling media) to form a slurry that can be deposited to a CMC substrate. The sintering aids have two primary functions: 1) densifying deposited slurry by generating liquid phases via reactions with the coating material and other sintering aids, so that the liquid fills gaps between particles of coated material; 2) enhancing bonding and performance of the coating by generating reaction products that enhance those qualities. One great advantage of this EBC is that it can be fabricated via various low-cost methods - including dipping, spinning, spin-dipping, painting, and spraying - in addition to plasma-spraying. Glenn's innovation rises to meet the need for a new class of EBCs that can keep up with CMCs' increasing ability to withstand higher temperatures and stresses than ever before.
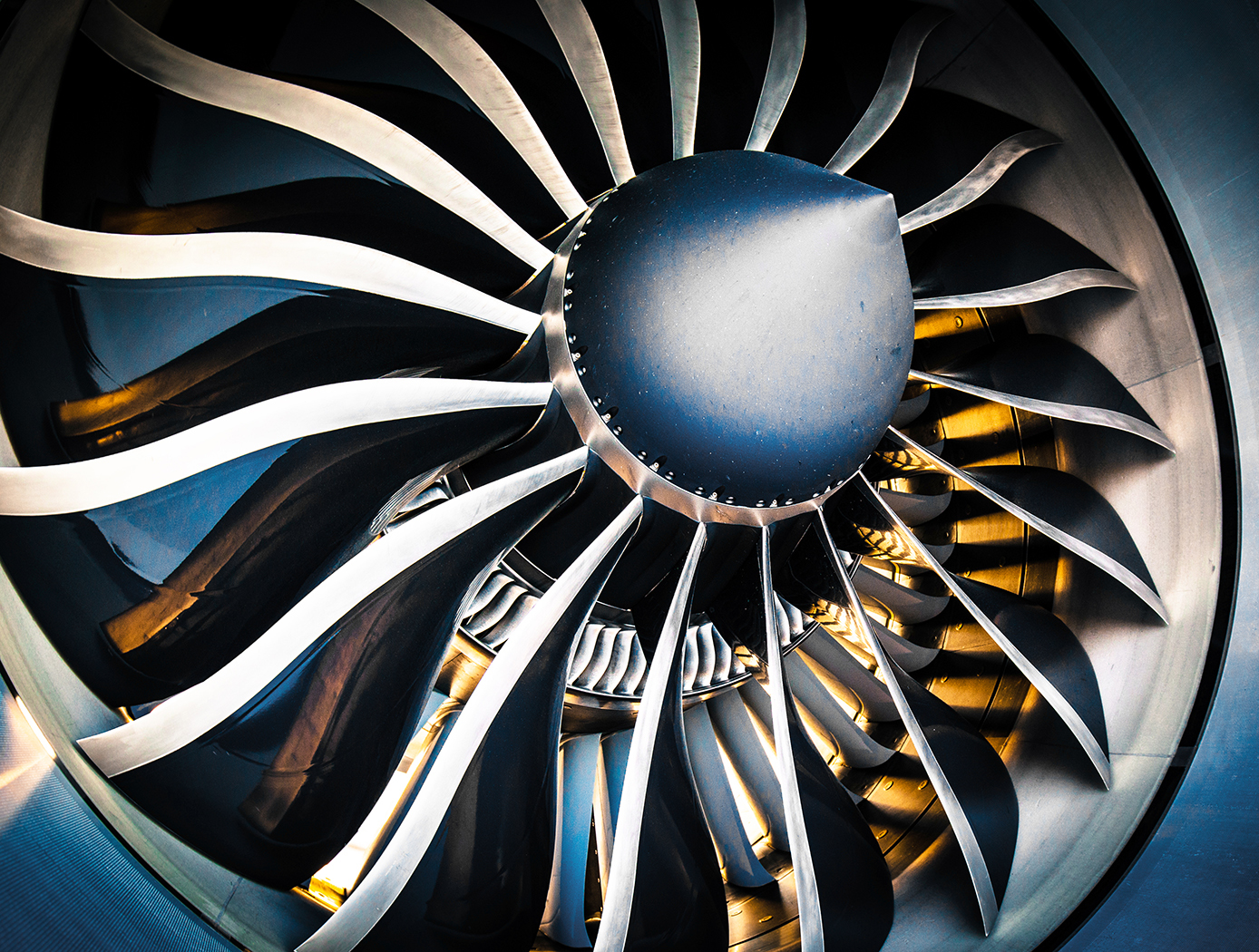
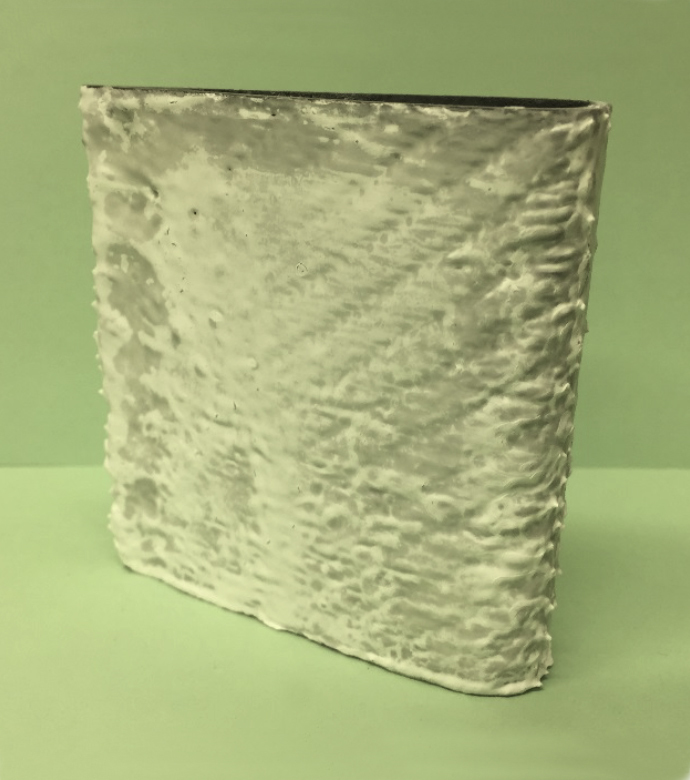
Benefits
- Durable: Maintains protection at unprecedented high temperatures
- Oxidation-resistant: Protects CMC components from most common causes of failure, water vapor and corrosive gases
- Easy to manufacture: Process allows liquid phase sintering to facilitate strong bonding
- Long-lasting: Proven steam oxidation life of at least 500 hours at 1482°C
- Cost-saving: Allows CMCs to remain in use for longer periods without replacement
Applications
- Aerospace
- Chemical manufacturing
- Heat exchangers
- Furnaces
- Power
- Thermal management
- Turbines (e.g. blades, vanes, combustor liners, bladetracks, shrouds, seals)
Similar Results

Silicon Carbide (SiC) Fiber-Reinforced SiC Matrix Composites
Aimed at structural applications up to 2700°F, NASA's patented technologies start with two types of high-strength SiC fibers that significantly enhance the thermo-structural performance of the commercially available boron-doped and sintered small-diameter “Sylramic” SiC fiber. These enhancement processes can be done on single fibers, multi-fiber tows, or component-shaped architectural preforms without any loss in fiber strength. The processes not only enhance every fiber in the preforms and relieve their weaving stresses, but also allow the preforms to be made into more shapes. Environmental resistance is also enhanced during processing by the production of a protective in-situ grown boron-nitride (iBN) coating on the fibers. Thus the two types of converted fibers are called “Sylramic-iBN” and “Super Sylramic-iBN”.
For high CMC toughness, two separate chemical vapor infiltration (CVI) steps are used, one to apply a boron nitride coating on the fibers of the preform and the other to form the SiC-based matrix. The preforms are then heat treated not only to densify and shrink the CVI BN coating away from the SiC matrix (outside debonding), but also to increase its creep resistance, temperature capability, and thermal conductivity.
One crucial advantage in this suite of technologies lies in its unprecedented customizability. The SiC/SiC CMC can be tailored to specific conditions by down-selecting the optimum fiber, fiber coating, fiber architecture, and matrix materials and processes. In any formulation, though, the NASA-processed SiC fibers display high tensile strength and the best creep-rupture resistance of any commercial SiC fiber, with strength retention to over 2700°F.

Thermal protection supplement for reducing interface thermal mismatch
The invention includes an exposed surface cap with a specially formulated coating, an insulator base adjacent to the cap with another specially formulated coating, and one or more pins that extend from the cap through the insulator base to tie the cap and base together through ceramic bonding and mechanical attachment. The cap and insulator base have corresponding depressions and projections that mate and allow for differences in thermal expansion of the cap and base. The cap includes a high-temperature, low density, carbonaceous, fibrous material whose surface is optionally treated with a High Efficiency Tantalum-based Ceramic Composite (HETC) formulation, the fibrous material being drawn from the group consisting of silicon carbide foam and similar porous, high temperature materials. The insulator base and pin(s) contain similar material. The mechanical design is arranged so that thermal expansion differences in the component materials (e.g., cap and insulator base) are easily tolerated. It is applicable to both sharp and blunt leading edge vehicles. This extends the possible application of fibrous insulation to the wing leading edge and/or nose cap on a hypersonic vehicle. The lightweight system comprises a treated carbonaceous cap composed of Refractory Oxidation-resistant Ceramic Carbon Insulation (ROCCI), which provides dimensional stability to the outer mold line, while the fibrous base material provides maximum thermal insulation for the vehicle structure. The composite has graded surface treatments applied by impregnation to both the cap and base. These treatments enable it to survive in an aero-convectively heated environment of high-speed planetary entry. The exact cap and base materials are chosen in combination with modified surface treatments and a specially formulated surface coating, taking into account the duration of exposure and expected surface temperatures for the particular application.

Layered Composite Insulation for Extreme Conditions (LCX)
The approach in developing the LCX system was to provide a combination of advantages in thermal performance, structural capability, and operations. The system is particularly suited for the complex piping, tanks, and apparatus subjected to the ambient environment common in the aerospace industry. The low-cost approach also lends the same technology to industrial applications such as building construction and chilled-water piping. The system can increase reliability and reduce life cycle costs by mitigating moisture intrusion and preventing the resulting corrosion that plagues subambient-temperature insulation systems operating in the ambient (humidity and rain) environment. Accumulated internal water is allowed to drain and release naturally over the systems normal thermal cycles. The thermal insulation system has a long life expectancy because all layer materials are hydrophobic or otherwise waterproof. LCX systems do not need to be perfectly sealed to handle rain, moisture accumulation, or condensation.
Mechanically, the LCX system not only withstands impact, vibration, and the stresses of thermal expansion and contraction, but can help support pipes and other structures, all while maintaining its thermal insulation effectiveness. Conventional insulation systems are notoriously difficult to manage around pipe supports because of the cracking and damage that can occur. Used alone or inside another structure or panel, the LCX layering approach can be tailored to provide additional acoustic or vibration damping as a dual function with the thermal insulating benefits. Because LCX systems do not require complete sealing from the weather, it costs less to install. The materials are generally removable, reusable, and recyclable, a feature not possible with other insulation systems. This feature allows removable insulation covers for valves, flanges, and other components (invaluable benefits for servicing or inspection) to be part of original designs.
Thermal performance of the LCX system has been shown to equal or exceed that of the best polyurethane foam systems, which can degrade significantly during the first two years of operation. With its inherent springiness, the system allows for simpler installation and, more importantly, better thermal insulation because of its consistency and full contact with the cold surface. Improved contact with the cold surface and better closure of gaps and seams are the keys to superior thermal performance in real systems. Eliminating the requirement for glues, sealants, mastics, expansion joints, and vapor barriers provides dramatic savings in material and labor costs of the installed system.

Vertically Aligned Carbon Nanotubes
Formation of the inventive polymer composite matrix begins by growing carbon nanotubes directly on a veil substrate. The carbon nanotubes are grown from both sides of a non woven carbon fiber mat. The carbon nanotubes can be single or multi walled and can be grown to predetermined lengths. The veiled substrate is positioned between carbon fiber/ polymer prepreg layers such that the carbon nanotubes protrude into the reinforcement layers. The polymer composite matrix formed following curing of the resin exhibits improved interlaminar strength, fracture toughness and impact resistance. Because of the thinness of the veil layer, electricity can pass from conductive carbon nanotubes on one side of the veil to conductive carbon nanotubes on the other side of the veil. Electricity can also pass between two veils intercalated into the same reinforcement layer when the length of the nanotubes is sufficiently long enough to provide overlap within the reinforcement layers.

Compact, Lightweight, CMC-Based Acoustic Liner
NASA researchers are extending an existing oxide/oxide CMC sandwich structure concept that provides mono-tonal noise reduction. That oxide/oxide CMC has a density of about 2.8 g/cc versus the 8.4 g/cc density of a metallic liner made of IN625, thus offering the potential for component weight reduction. The composites have good high-temperature strength and oxidation resistance, allowing them to perform as core liners at temperatures up to 1000°C (1832°F). NASA's innovation uses cells of different lengths or effective lengths within a compact CMC-based liner to achieve broadband noise reduction. NASA has been able to optimize the performance of the proposed acoustic liner by using improved design tools that help reduce noise over a specified frequency range. One such improvement stems from the enhanced understanding of variable-depth liners, including the benefits of alternate channel shapes/designs (curved, bent, etc.). These new designs have opened the door for CMC-based acoustic liners to offer core engine noise reduction in a lighter, more compact package. As a first step toward demonstrating advanced concepts, an oxide/oxide CMC acoustic testing article with different channel lengths was tested. Bulk absorbers could also be used, either in conjunction with or in place of the liners internal chambers, to reduce noise further if desired.