3D-Printed Composites for High Temperature Uses
materials and coatings
3D-Printed Composites for High Temperature Uses (LEW-TOPS-145)
Using Laser Sintering to Manufacture Thermoset Polyimide Composites
Overview
Innovators at the NASA Glenn Research Center, in collaboration with the University of Louisville and the U.S. Air Force, have developed an additive manufacturing technique to produce composite parts with high-temperature capabilities using thermoset polyimide resins. The process uses selective laser sintering (SLS) to melt-process a powdered version of NASA's novel RTM370 imide resin filled with finely milled carbon fibers. The resulting composite part can be subsequently post-cured to prepare for high temperature aerospace applications, offering a 3D-printed composite part that can withstand temperatures over 300 °C. This is a significant advancement in the state-of-the-art in additive manufacturing polymers, offering an SLS process that requires relatively low melting temperatures and creates composites with high temperature capabilities, enabling 3D-printing of parts with complex geometry for high performance applications.
The Technology
NASA's technology is the first successful 3D-printing of high temperature carbon fiber filled thermoset polyimide composites. Selective Laser Sintering (SLS) of carbon-filled RTM370 is followed by post-curing to achieve higher temperature capability, resulting in a composite part with a glass transition temperature of 370 °C.
SLS typically uses thermoplastic polymeric powders and the resultant parts have a useful temperature range of 150-185 °C, while often being weaker compared to traditionally processed materials. Recently, higher temperature thermoplastics have been manufactured into 3D parts by high temperature SLS that requires a melting temperature of 380 °C, but the usable temperature range for these parts is still under 200 °C.
NASA's thermoset polyimide composites are melt-processable between 150-240 °C, allowing the use of regular SLS machines. The resultant parts are subsequently post-cured using multi-step cycles that slowly heat the material to slightly below its glass transition temperature, while avoiding dimensional change during the process. This invention will greatly benefit aerospace companies in the production of parts with complex geometry for engine components requiring over 300 °C applications, while having a wealth of other potential applications including, but not limited to, printing legacy parts for military aircraft and producing components for high performance electric cars.
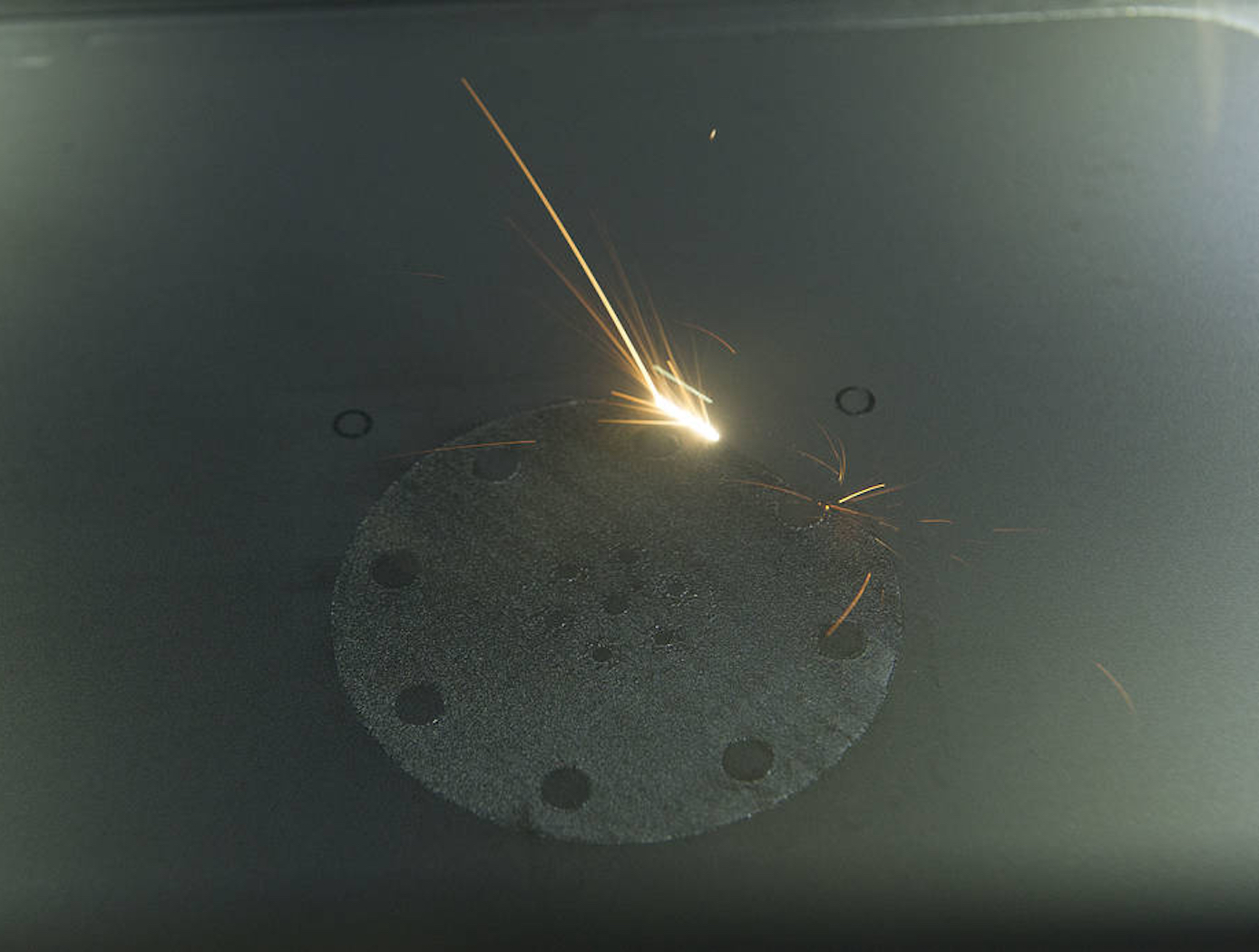
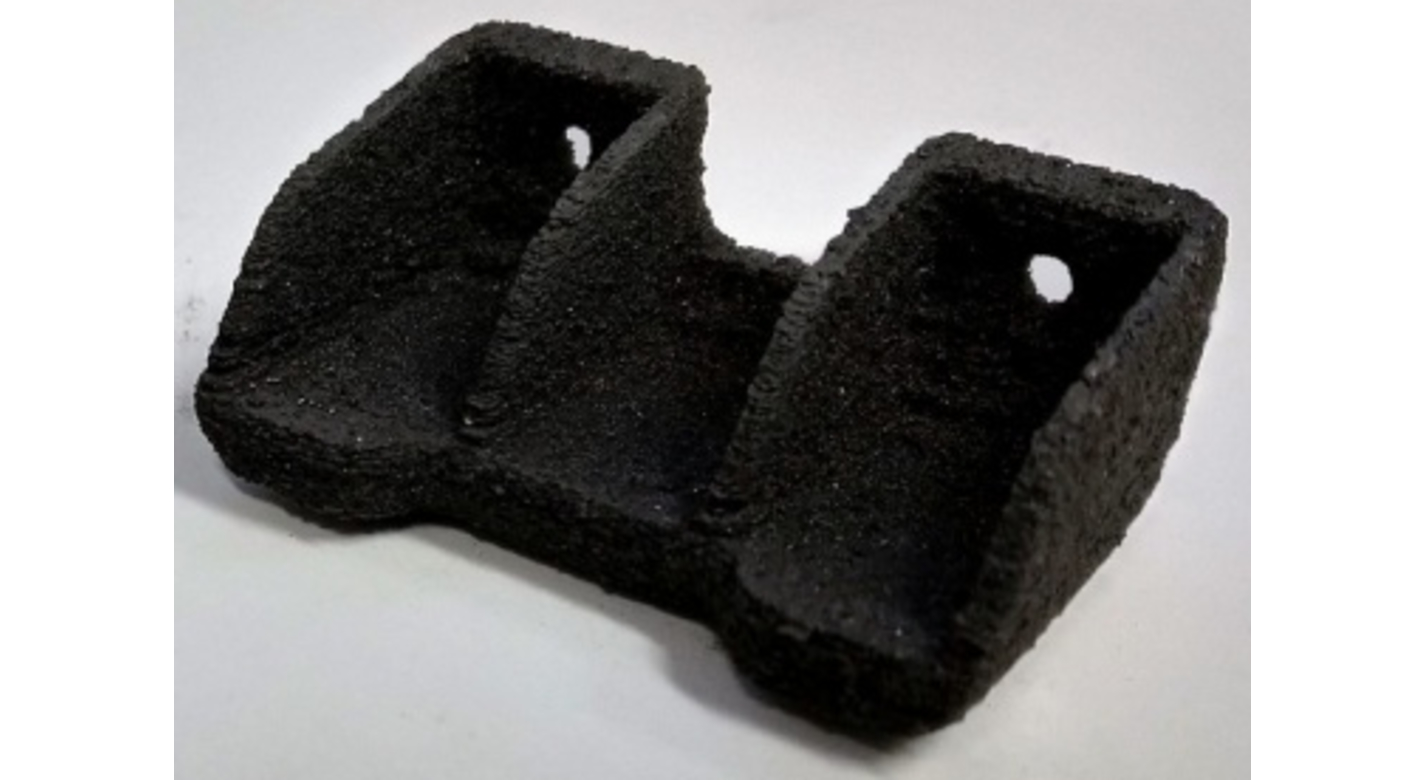
Benefits
- High-temperature capability: NASA's thermoset polyimide composites retain mechanical properties at extremely high temperatures (over 300 °C)
- Simple production of high-performance, complex 3D parts: Objects with complicated structures that require high temperature applications can be 3D-printed by a regular SLS machine
- Lightweight components: Parts made from RTM370 composites are 30 percent lighter than metallic parts
- Clean and green: RTM370 uses a solvent-free production process that does not produce any harmful, volatile compounds
- Excellent impact-resistance and char yield: RTM370 composites demonstrate high impact resistance and outstanding abrasion resistance at ambient and high temperatures
Applications
- Aerospace
- Automotive
- Commercial space
- Construction
- Electronics
- Industrial machinery
- Marine
- Mechanical systems
- Oil and gas
Technology Details
materials and coatings
LEW-TOPS-145
LEW-19873-1
LEW-17618-1
"Laser Sintering of Thermoset Polyimide Composites," Kathy C. Chuang, et al., September 23, 2019,
https://ntrs.nasa.gov/search.jsp?R=20190031841.
https://ntrs.nasa.gov/search.jsp?R=20190031841.
Tags:
|
Similar Results

Resin Transfer Molding (RTM) 370 Resin for High-Temperature Applications
RTM370 imide resin was developed to address the limitations of conventional imide resins, which are generated from commercially available symmetrical biphenyl dianhydride and oxydianiline (ODA). These resins form symmetrical dianhydride or diamine compounds that result in a substance with much higher viscosity than is viable for RTM, RFI, and VARTM. RTM370 harnesses the unique properties of asymmetric biphenyl dianhydride (a-BPDA) used in combination with a kinked ODA and a 4-(Phenylethynyl) phthalic anhydride endcap to form a mixture that can be melted without the use of solvents, and achieve the desired low-melt viscosity. RTM370 displays a high softening temperature (Tg = 370°C) and can be melted at 260-280°C. It can then be injected into fiber preforms under pressure (200 psi) or through a vacuum (VARTM) to form composites with excellent toughness. The resin can also be made into powder prepregs by melting the resin powders so that they fuse onto fibers. Recently, carbon fiber filled RTM370 imide resins have been fabricated into composites by laser sintering. This exciting advancement in additive manufacturing represents a new frontier for high-temperature composites.
Not only are RTM370 composites lightweight, durable, and impact-resistant, they also possess outstanding abrasion resistance and significant thermo-oxidative stability (as demonstrated in long-term isothermal aging at 288°C for 1,000 hours). In summary, this groundbreaking approach yields a vastly superior resin for fabricating high-quality composites with improved performance, durability, and adaptability. RTM370's unique, solvent-free melt process is simpler, more environmentally friendly, and more cost-effective than competing systems, lending it broad appeal for a variety of Earth-based applications.

Automated Tow/Tape Placement System
This NASA invention enables several benefits that mitigate limitations associated with conventional ATP systems, including the following: (1) avoids obtuse head rotation or cross-tool translation when laying adjunct tape plies, (2) simultaneously places tape on both sides of a part via two robots, (3) eliminates external anchoring frame requirements, and (4) translates parts during build while also translating the applicator head. The ability to perform simultaneous layup on opposite sides of the component, as well as reduction of head rotation reversal during bidirectional tape layup, offers increased layup speed. The invention offers increased placement accuracy as a result of reduced movement between tape layup operations and the eliminated need for an anchoring frame (facilitated by simultaneous pressure extrusion of prepreg by the two robots).
NASAs automated tow/tape placement system has two key unique features: the use of two opposed ATP cars to enable a tool-less process, and an on-the-fly reversal tape/tow laydown tooling head. The system uses two opposing (i.e., underside-to-underside) ATP cars, and can build parts vertically, horizontally, or at any other angle, depending on the workspace available. The ATP die wheels can be reversed or turned to draw the composite back and forth at different angles to create a layer-by-layer composite structure. Both cars can dispense TPC tape thus, either car can function as an opposing tool surface while the other performs prepreg lay-up. For structures that do not vary in thickness, both cars can lay tape at the same time doubling layup speed. Current ATP robots must rotate the large tooling head, or traverse panels without layering tape to achieve bidirectional layup, where each additional movement introduces alignment error. To increase layup rate while simultaneously minimizing misalignment, NASAs system incorporates an on-the-fly reversal tape/tow laydown tooling head to enable efficient bidirectional layup.

A New Class of Strong and Flexible Carbon Fiber Reinforced Phenolic Composites
This unique approach modifies the phenolic polymer network by adding thermoplastic molecules with flexible segments such as aliphatic carbon and siloxane. The thermoplastic molecules are terminated with bifunctional groups that can directly react with the phenolic under the curing condition to form chemical bonds. Further incorporation of these segments can be facilitated by a relay reaction of a second molecular component which can bond with both the first flexible segments and the phenolic network. The selections of flexible, thermoplastic segments are based on desired properties, which include flexibility, ablative, an charring ability, heat resistance, and low catalycity.
The modified phenolic is a truly molecular composite in which flexible segments are connected with the phenolic network through strong chemical bonds and are uniformly distributed among the networks. This feature renders a uniform toughening/strengthening effect without compromising the lightweight nature of the materials. The process is also feasible to scale up and amenable for manufacturing.

A One-piece Liquid Rocket Thrust Chamber Assembly
The one-piece multi-metallic composite overwrap thrust chamber assembly is centrally composed of an additively manufactured integral-channeled copper combustion chamber. The central chamber is being manufactured using a GRCop42 or GRCop84 copper-alloy additive manufacturing technology previously developed by NASA. A bimetallic joint (interface) is then built onto the nozzle end of the chamber using bimetallic additive manufacturing techniques. The result is a strong bond between the chamber and the interface with proper diffusion at the nozzle end of the copper-alloy. The bimetallic interface serves as the foundation of a freeform regen nozzle. A blown powder-based directed energy deposition process (DED) is used to build the regen nozzle with integral channels for coolant flow. The coolant circuits are closed with an integral manifold added using a radial cladding operation. To complete the TCA, the entire assembly including the combustion chamber and regen nozzle is wrapped with a composite overwrap capable of sustaining the required pressure and temperature loads.

Silicon Carbide Fiber Tows
SiC fiber tows and preform materials are commonly used as reinforcements in CMCs, to make parts for use in harsh, high-temperature environments such as aircraft engines. These materials are desirable for numerous high-temperature applications because of their very low weight and outstanding thermo-chemical inertness. However, the multiple-step process using electric furnaces to produce these materials have numerous drawbacks: they are very expensive ($10,000 to $25,000 per spool); they involve high temperatures (greater than 2000°C); they require high power (more than 700 watts); and they produce much wasted material. Glenn innovators have discovered an efficient way to improve the quality and strength of SiC fiber tows using a unique microwave-furnace design that induces molecular heating. Glenn's innovation relies on microwave sintering to convert a polymer to ceramic fibers/tows/yarns, or to manipulate commercially available SiC fibers to increase strength and improve other qualities. Not only can higher quality tows be produced, but also - for the first time - old, damaged, or otherwise unusable fibers can be improved and recycled, thereby saving significant costs by increasing yield. Even entire engine components can be placed in the furnace and restored. The desired results can be achieved in minutes rather than the usual hours or even days. Glenn's low-temperature microwave process provides greater control with less power, while also eliminating plasma generation and minimizing arcing events. Because this method also facilitates the shaping of the SiC fiber after initial processing, fabricating preforms with 2D or 3D architectures becomes simpler. Glenn's creative processing method makes producing SiC tows and preforms much less expensive, opening them up for increased use in a broad range of applications.