Oxide Dispersion Strengthened Medium Entropy Alloy
Materials and Coatings
Oxide Dispersion Strengthened Medium Entropy Alloy (LEW-TOPS-151)
An Additively Manufactured Alloy Tailored for High-Temperature Applications
Overview
Innovators at the NASA Glenn Research Center have developed a new oxide dispersion strengthened medium entropy alloy (ODS-MEA) using additive manufacturing (AM). ODS alloys, in which nano-scale ceramic particles are distributed within the metal, were originally developed to enhance mechanical properties (e.g., creep resistance, tensile strength, microstructure integrity) at extreme temperatures. Thus, such alloys show promise for metal components of gas turbines, rocket engines, nuclear reactors and other high-temperature applications. However, the conventional mechanical alloying process to produce such alloys is highly inefficient, time-consuming, and costly. By contrast, NASA's ODS-MEA is designed for production via selective laser melting. The alloy can be fabricated into complex geometries and is resistant to stress cracking and dendritic segregation. It is not susceptible to deleterious phase changes when exposed to extreme temperatures and requires limited post-processing.
The Technology
NASA's ODS-MEA maintains properties up to 1100°C and is not susceptible to deleterious phase changes when exposed to extreme temperatures, an issue ubiquitous to Ni- based superalloys such as Inconel-625 and Inconel-718. Yttria particles are dispersed throughout the alloy to maximize strength and creep resistance at high temperatures using a novel fabrication technique. This technique employs an acoustic mixer to stir nano-scale Yttria oxide powder within a metallic matrix powder, creating a film of Yttria surrounding the larger metallic powder particles. Solid components are then produced from this mixture via SLM, during which the laser disperses the Yttria particles throughout the microstructure. Ultimately, the process eliminates the many expensive and time-consuming steps in the production of ODS alloys via traditional mechanical alloying. NASA's process has been shown to fabricate components with 10x improvement in creep rupture life at 1100°C and provides a 30% increase in strength over what is currently possible with 3D printed parts. The new ODS-MEA composition may find applications where ODS alloys are currently used (e.g., those involving extreme thermal environments). Applications may also include areas where such properties are desirable but the resource-intensive nature and/or inability to produce highly complex geometries via conventional processes ultimately renders their use uneconomical or infeasible. Such uses include gas turbine components (for which increasing inlet temperature enables improved efficiency) for power generation, propulsion (rockets, jet engines, etc.), industrial processes, nuclear energy applications, and sample preparation equipment in the mining and cement production industries, among many others.
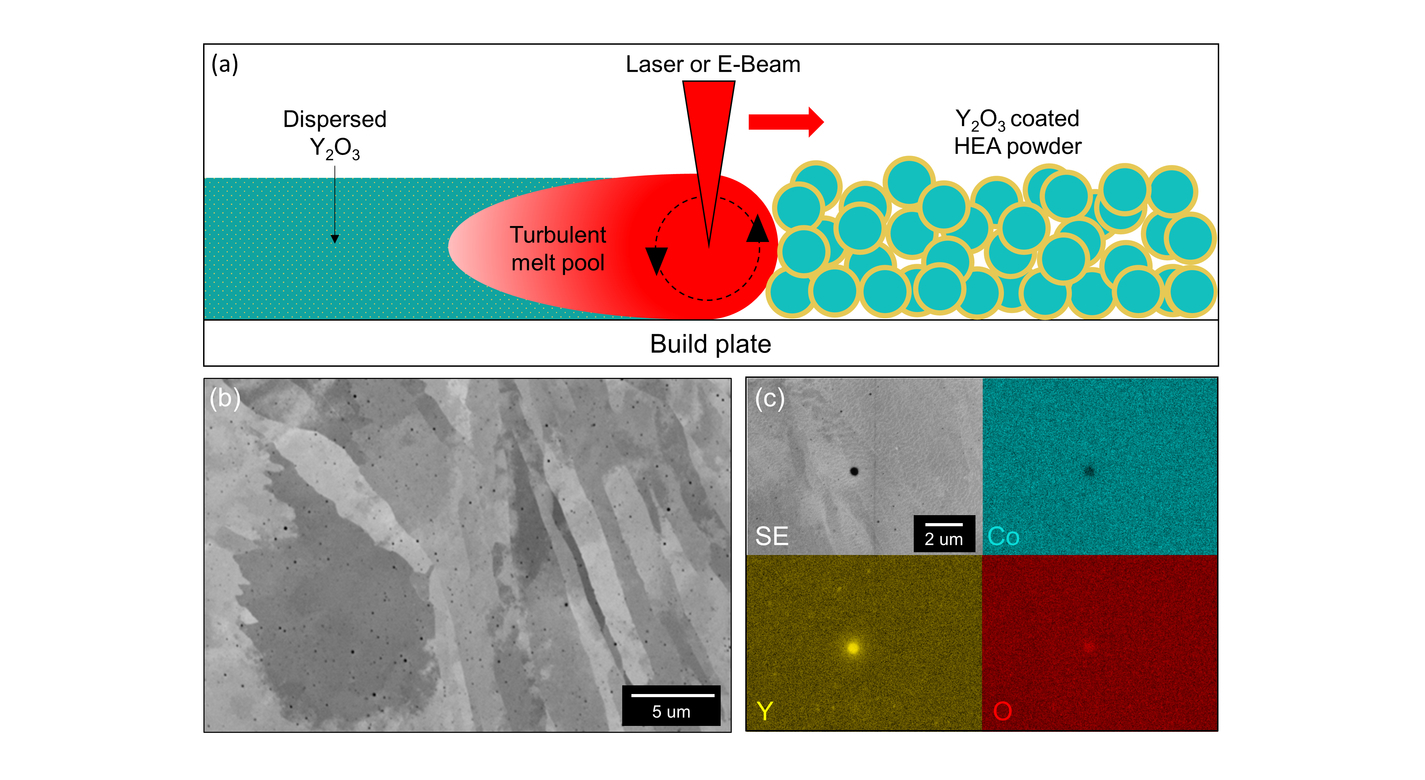
Benefits
- Maintains properties up to 1100°C: the alloy is not susceptible to deleterious phase changes when exposed to extreme temperature environments (a common issue for Ni-based superalloys), and is resistant to stress cracking and dendritic segregation
- Highly efficient process: eliminates numerous expensive and time-consuming steps for producing ODS alloys via conventional mechanical alloying processes and requires limited post-processing
- Adaptable, improved fabrication: the oxide-based process has been shown to fabricate components with 10x improvement in creep rupture life at 1100°C and a 30% increase in strength relative to current 3D-printable metal parts
Applications
- Aerospace: high-temperature components for space launch systems and jet turbine engines
- Industrial machinery: chemical processing and waste processing systems
- Marine: turbine engines for ships
- Oil and gas: oil refining process
- Power: steam turbines and gas turbines for electricity generation, structural components for solar thermal power plants, heat exchangers for nuclear reactor systems
- Propulsion: rockets, jet engines, etc.
Technology Details
Materials and Coatings
LEW-TOPS-151
LEW-19886-1
LEW-20020-1
"Microstructural and Mechanical Characterization of a Dispersion Strengthened Medium Entropy Alloy Produced Using Selective Laser Melting," Smith, T.M., et al., November 17, 2019, https://ntrs.nasa.gov/citations/20200000360
Tags:
|
Similar Results

Innovative Shape Memory Metal Matrix Composites
Shape memory alloys (SMAs) are metals that can return to their original shape following thermal input. They are commonly used as functional materials in sensors, actuators, clamping fixtures and release mechanisms across industries. SMAs can suffer from dimensional/thermal instability, creep, and/or low hardness, resulting in alloys with little to no work output in the long term. To combat these deficiencies, NASA has developed a process of incorporating nanoparticles of refractory materials (i.e., carbide, oxide, and nitride materials with high temperature resistance) into the alloys.
Using various processing methods, the nanoparticles can be effectively mixed and dispersed into the metal alloys as shown in the figure below. In these processes the SMA and refractory material powder is mixed and the refractory nanoparticles incorporated through extrusions, melting, or directly used in additive manufacturing to create parts for applications across the aerospace, automotive, marine, or biomedical sectors. The nanoparticle dispersion is a controllable method to strengthen the SMAs, increasing the hardness of the alloys, reducing the impact of creep, and improving the overall dimensional and thermal stability of the alloys.
The related patent is now available to license. Please note that NASA does not manufacture products itself for commercial sale.

Shape Memory Alloy with Adjustable, Wide-Ranging Actuation Temperatures
SMAs are important multifunctional materials for the development of adaptive engineering structures. They exhibit a high work output that is competitive with, or superior to, conventional hydraulic, pneumatic, or electromagnetic actuators. While highly promising, SMAs are not always a practical alternative to conventional actuators because of their limited phase transformation temperatures and dimensional instability. Thanks to Glenn's innovative new SMA, that's about to change.
Unlike traditional binary NiTi SMAs, Glenn's Ni-Ti-Hf-Zr SMA includes secondary, nanoscale precipitate phases that offer inherent dimensional stability to the material. Consequently, there is minimal to no need for training, resulting in much faster production times, lower processing costs, and a finished product with superior work outputs and better operational life. These Ni-rich alloys can be produced by Vacuum Induction Melting, Vacuum Arc Melting, Vacuum Arc Remelting, and Induction Skull Melting. Perhaps the most exciting characteristic of Glenn's SMA, however, is its ability to achieve a broad range of transformation temperatures suitable for high temperature (100 to 300°C), ambient, and sub-ambient temperature applications nearing -100°C. Furthermore, these temperatures can be tailored and fine-tuned though heat treatment to fit the needed parameters for the application of interest. In contrast, traditional NiTi SMAs exhibit fixed phase transformation at temperatures from slightly below room temperature to around 100°C. Glenn's Ni-Ti-Hf-Zr SMA opens the door to countless applications that can benefit from the unique properties of SMAs but require high durability and extreme temperature capability.

3D-Printed Composites for High Temperature Uses
NASA's technology is the first successful 3D-printing of high temperature carbon fiber filled thermoset polyimide composites. Selective Laser Sintering (SLS) of carbon-filled RTM370 is followed by post-curing to achieve higher temperature capability, resulting in a composite part with a glass transition temperature of 370 °C.
SLS typically uses thermoplastic polymeric powders and the resultant parts have a useful temperature range of 150-185 °C, while often being weaker compared to traditionally processed materials. Recently, higher temperature thermoplastics have been manufactured into 3D parts by high temperature SLS that requires a melting temperature of 380 °C, but the usable temperature range for these parts is still under 200 °C.
NASA's thermoset polyimide composites are melt-processable between 150-240 °C, allowing the use of regular SLS machines. The resultant parts are subsequently post-cured using multi-step cycles that slowly heat the material to slightly below its glass transition temperature, while avoiding dimensional change during the process. This invention will greatly benefit aerospace companies in the production of parts with complex geometry for engine components requiring over 300 °C applications, while having a wealth of other potential applications including, but not limited to, printing legacy parts for military aircraft and producing components for high performance electric cars.

High-Temperature Ni-Based Superalloy Composition
NASA's new Ni-based superalloy uses a powder metallurgy (PM) composition that inhibits the deleterious gamma-prime to gamma-phase transformation along stacking faults during high temperature creep deformation. Ni-base superalloys have excellent high temperature properties, mostly due to the presence of coherent precipitates. At higher temperatures, these precipitates are defeated by the diffusional shear dislocations producing intrinsic and extrinsic faults. Recent studies have found that, during deformation of turbine disk alloys at high temperature, Co, Cr, and Mo segregate to these faults (removing Ni and Al) inside the strengthening precipitates of these alloys. This represents a local phase transformation from the strengthening precipitate to the weaker matrix phase. Therefore, this elemental segregation significantly weakens the ability of a precipitate to withstand further deformation, producing faster strain rates in the alloy at higher temperatures. This invention presents a solution to prevent this type of segregation along these two faults to improve the creep properties of turbine disks and similar Ni-based alloys. By alloying a specific amount of eta phase formers (Ti, Ta, Nb, and Hf), the phase transformation to can be eliminated along 2-layer extrinsic stacking faults (SESFs) in precipitates without precipitating bulk eta phase. Also, by adding a certain amount of D019 formers (Mo and W), the phase transformation to can be mitigated along 1-layer intrinsic stacking faults (SISFs) without producing bulk sigma phase. This alloy composition incorporates both strengthening methods for use in jet turbine disks, though the composition has applications in other high-stress and/or high-temperature environments as are found in power plants, space launch systems, and other critical structural applications.

Silicon Carbide (SiC) Fiber-Reinforced SiC Matrix Composites
Aimed at structural applications up to 2700°F, NASA's patented technologies start with two types of high-strength SiC fibers that significantly enhance the thermo-structural performance of the commercially available boron-doped and sintered small-diameter “Sylramic” SiC fiber. These enhancement processes can be done on single fibers, multi-fiber tows, or component-shaped architectural preforms without any loss in fiber strength. The processes not only enhance every fiber in the preforms and relieve their weaving stresses, but also allow the preforms to be made into more shapes. Environmental resistance is also enhanced during processing by the production of a protective in-situ grown boron-nitride (iBN) coating on the fibers. Thus the two types of converted fibers are called “Sylramic-iBN” and “Super Sylramic-iBN”.
For high CMC toughness, two separate chemical vapor infiltration (CVI) steps are used, one to apply a boron nitride coating on the fibers of the preform and the other to form the SiC-based matrix. The preforms are then heat treated not only to densify and shrink the CVI BN coating away from the SiC matrix (outside debonding), but also to increase its creep resistance, temperature capability, and thermal conductivity.
One crucial advantage in this suite of technologies lies in its unprecedented customizability. The SiC/SiC CMC can be tailored to specific conditions by down-selecting the optimum fiber, fiber coating, fiber architecture, and matrix materials and processes. In any formulation, though, the NASA-processed SiC fibers display high tensile strength and the best creep-rupture resistance of any commercial SiC fiber, with strength retention to over 2700°F.