Robust Sensors Detect Material Ablation and Temperature Changes
sensors
Robust Sensors Detect Material Ablation and Temperature Changes (LEW-TOPS-83)
Embedded and arrayed sensors enable large-area sensing in thermal protection systems and more
Overview
Innovators at NASA's Glenn Research Center developed a microsensor suite to measure real-time changes in thickness and temperature on the surface of a material undergoing ablation. Corrosion, ablation, attrition, and erosion are examples of material damage that affects surface integrity and product performance and could compromise safety of lives and property. The ability to reliably and efficiently monitor the temperature and rate at which surface materials deteriorate is important, especially for safety critical systems. Originally designed for use in thermal protection systems (TPS) of space vehicles, these embedded and distributed sensors foster large-area sensing. Glenn's development also holds great potential for monitoring accelerated attrition rate caused by the high velocity flow of liquid-sand or other mixtures through pipes. Real-time monitoring of these conditions will enable optimal product performance before replacement.
The Technology
Glenn's breakthrough technology introduces batch-fabricated, miniature sensors embedded and distributed over a large surface area of a material or product during the manufacturing process. The sensors can be utilized for test instrumentation or as an integrated in-situ monitoring system. This integrated manufacturing approach preserves the structural and mechanical system integrity by eliminating the antiquated plug-in approach, invasive machining, manual insertion, and gluing processes currently required to implant sensors into a material. The sensor ladder network of resistors and capacitors breaks down as result of the thermo-physical effects caused by temperature, shock, radiation, corrosion, or other reactions, causing a change in the electrical properties. A processor interprets these changes in the electrical properties and generates a high-resolution, large-area surface profile. The profile demonstrates the amount or rate of material deterioration and temperature change, and is used to optimize geometric structural design, develop materials, predict performance, and make decisions. These sensors play an important role as industries work to realize material performance and product design. This type of monitoring is ideal for infrastructures, nuclear enclosures, or any system susceptible to surface deterioration.
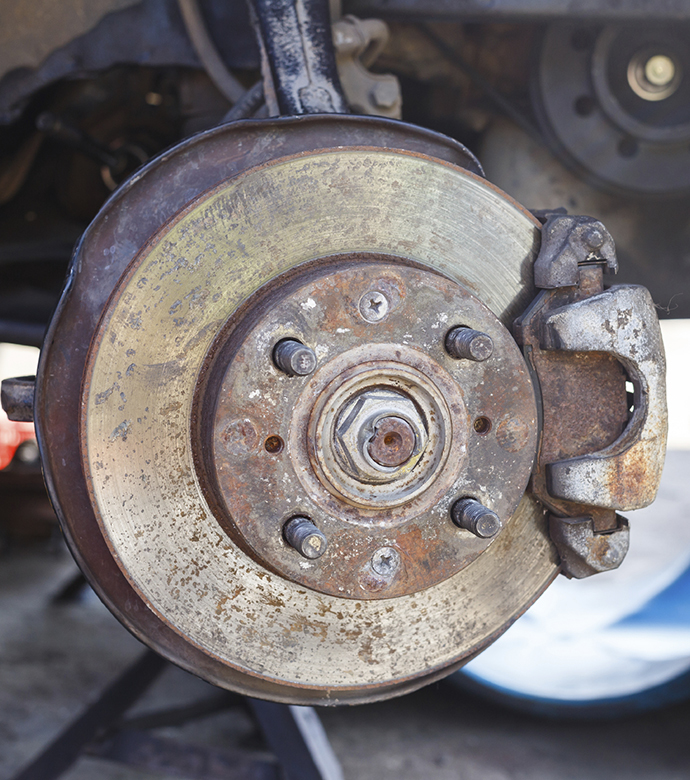
Benefits
- Efficient: Embeds sensors and telemetry during the manufacturing process, eliminating the expensive and time-consuming practice of manually inserting thermocouples and resistors into materials
- Versatile: Allows numerous sensors to be implanted for high-resolution/large-area profiling
- Compact: Allows for miniaturized, lightweight, micron scale sensor networks
- Reliable: Optimizes material and product development to increase performance and prioritize safety
- Economical: Batch fabrication results in lower costs
Applications
- Vehicles (braking systems)
- Thermal protection systems (space vehicles, missiles, hyper-loop vessels)
- Oil and gas (pipe erosion)
- System monitoring (nuclear containment, infrastructure erosion)
Similar Results

Polymer Electrolyte-Based Ambient Temperature Oxygen Microsensor
Conventional ambient-temperature oxygen sensors are limited in various ways: optically based sensors can be expensive and challenging to manufacture; electrochemical cells with liquid electrolytes can have limited lifetimes and become leak sources; and both types of sensors are difficult to miniaturize. These problems are addressed with Glenn's novel ambient temperature oxygen microsensor, which is based on a Nafiontm polymer electrolyte, microfabricated using thin-film technologies. In the past, one drawback of Nafiontm film has been that it can lose conductivity when the moisture content in the film is too low, potentially affecting sensor operation. Glenn researchers devised a method to use certain salts to hold water molecules in the Nafiontm film structure at room temperature. The presence of these salts provides extra sites in the film to promote proton (H+) mobility, thus improving film conductivity and overall sensor performance, particularly in arid and high-temperature environments.
The innovative use of metal/metal oxide as the reference electrode enables miniaturization by eliminating the reference gas and sealing the reference electrode. The combination of interdigitized electrodes with the unique metal/metal oxide reference electrode permits sensor operation in either potentiometric or amperometric mode, as appropriate. In potentiometric mode, which measures voltage differences between working and reference electrodes in different gases, the voltage differences can be monitored with a voltmeter; however, the sensor itself does not need a power source. In room-temperature testing, the sensor achieved repeatable responses to 21 percent oxygen in nitrogen (using nitrogen as a baseline gas), and also detected oxygen from 7 to 21 percent, making Glenn's breakthrough technology usable for personal health monitoring as well as fire detection, fuel-leak detection, and environmental monitoring.

Wireless Temperature Sensor Having No Electrical Connections
This technology is a new sensor made up of dielectric materials tuned to accurately measure a variable and wide range of temperatures. The sensor is wireless and is powered by an external magnetic field. As the temperature changes, the dielectric material changes its signature magnetic response and the change is detected by a magnetic field response sensor. Applications for this technology are temperature sensors for non-conductive surfaces where the conditions or operations require a robust and wireless sensor.

Packaging for SiC Sensors and Electronics
Prior approaches to bonding a SiC sensor and a SiC cover member relied on either electrostatic bonding or direct bonding using glass frits. The problem with the former is that its relatively weak bond strength may lead to debonding during thermal cycling, while the latter requires the creation of apertures that can allow sealant to leak. Glenn's innovation uses NASA's microelectromechanical system direct chip attach (MEMS-DCA) technology that can be bulk-manufactured to reduce sensor costs. The MEMS-DCA process allows a direct connection to be made between chip and pins, thereby eliminating wire bonding. Sensors and electronics are attached in a single-stage process to a multifunctional package, which, unlike previous systems, can be directly inserted into the housing. Additional thick pins within the electrical outlet allow the package to be connected to external circuitry. Furthermore, because the top and bottom substrates' thermomechanical properties are similar to that of the sensors, the problem of mismatch in the coefficient of thermal expansion is significantly reduced, minimizing thermal cycling and component fatigue. By protecting sensors and electronics in temperatures up to 600°C, approximately twice what has previously been achievable, Glenn's innovation enables SiC components to realize one of their most exciting possibilities - direct placement within high-temperature environments.

ShuttleSCAN 3-D
How It Works
The scanners operation is based on the principle of Laser Triagulation. The ShuttleSCAN contains an imaging sensor; two lasers mounted on opposite sides of the imaging sensor; and a customized, on-board processor for processing the data from the imaging sensor. The lasers are oriented at a given angle and surface height based on the size of objects being examined. For inspecting small details, such as defects in space shuttle tiles, a scanner is positioned close to the surface. This creates a small field of view but with very high resolution. For scanning larger objects, such as use in a robotic vision application, a scanner can be positioned several feet above the surface. This increases the field of view but results in slightly lower resolution. The laser projects a line on the surface, directly below the imaging sensor. For a perfectly flat surface, this projected line will be straight. As the ShuttleSCAN head moves over the surface, defects or irregularities above and below the surface will cause the line to deviate from perfectly straight. The SPACE processors proprietary algorithms interpret these deviations in real time and build a representation of the defect that is then transmitted to an attached PC for triangulation and 3-D display or printing. Real-time volume calculation of the defect is a capability unique to the ShuttleSCAN system.
Why It Is Better
The benefits of the ShuttleSCAN 3-D system are very unique in the industry. No other 3-D scanner can offer the combination of speed, resolution, size, power efficiency, and versatility. In addition, ShuttleSCAN can be used as a wireless instrument, unencumbered by cables. Traditional scanning systems make a tradeoff between resolution and speed. ShuttleSCANs onboard SPACE processor eliminates this tradeoff. The system scans at speeds greater than 600,000 points per second, with a resolution smaller than .001". Results of the scan are available in real time, whereas conventional systems scan over the surface, analyze the scanned data, and display the results long after the scan is complete.

Inexpensive Microsensor Fabrication Process
Because chemical sensors are used in many aspects of space missions, NASA researchers are continually developing ever smaller and more robust sensors that can be manufactured inexpensively and in high quantities; e.g., in batches. Glenn has developed a way to inexpensively fabricate microsensors using a sacrificial template approach. A nanostructure, such as a carbon nanotube, serves as a template, which can then be coated with a high-temperature oxide material. The carbon nanotube can be burned off, or sacrificed, leaving only the metal oxide. The resulting structure provides the unique morphology and properties of the carbon nanotube, which are advantageous for sensing, along with the material durability and high-temperature sensing capabilities of the metal oxide. This technique increases the surface area available for sensing because both the interior and exterior of the resulting microsensor can be used for gas detection, significantly increasing performance.
The fabrication of these microsensors includes three major steps: (1) synthesis of the porous metal or metal oxide nanostructures using a sacrificial template, (2) deposition of the electrodes onto alumina substrates, and (3) alignment of the nanostructures between the electrodes. The invention has been demonstrated for methane detection at room temperature (using tin oxide, with carbon nanotubes as the sacrificial template). The microsensor offers low power consumption (no heating required), compact size, extremely low cost, and simple batch-fabrication.