High-Temperature Single Crystal Preloader
mechanical and fluid systems
High-Temperature Single Crystal Preloader (LEW-TOPS-97)
Advanced single crystal spring preloaders maintain resiliency in excess of 2000°F
Overview
Many aerospace vehicles require thermal barriers/seals to minimize the flow of hot gases through interfaces in thermal protection systems and propulsion systems where temperatures can exceed 1200°F. One of the primary challenges is maintaining proper sealing contact at elevated temperatures. To address this challenge, preloading elements in various spring-like forms are often incorporated into or behind a seal to provide the required resiliency. At these elevated temperatures, conventional spring preloaders made from polycrystalline alloys lose strength and can exhibit excessive creep, resulting in a loss of sealing contact. Glenn innovators have developed new processes to enable the fabrication of preloaders made of single crystal superalloys that can increase the upper limit of thermal seals to greater than 2000°F for short to moderate term applications, and greater than 1800°F for long term applications. These preloaders can be manufactured in a variety of configurations allowing stiffness to be customized for a particular application.
The Technology
For extremely high-temperature sealing applications, Glenn researchers have devised novel methods for fabricating single-crystal preloaders. NASA's high-temperature preloaders consist of investment cast or machined parts that are fabricated in various configurations from single crystal superalloys. Machined preloaders include a variety of spring configurations, compressed axially or radially, fabricated from single crystal slabs. Before machining, the slabs are carefully oriented in a special goniometer using x-diffraction techniques. This helps to maintain proper crystal orientation relative to the machined part and the applied loads. For more complex geometry components which cannot be easily and economically machined, an investment casting approach would be used. Complex preloader geometries include wire coil springs of various configurations. These single crystal preloaders would be designed with the appropriate stiffness for the intended thermal barrier/seal application and placed underneath, or integrated within, the seal/barrier. At extrememly high temperature, the preload device keeps the seal/barrier mated against the opposing surface as the gap between the two surfaces changes, maintaining contact between surfaces and preventing convective heat transfer.
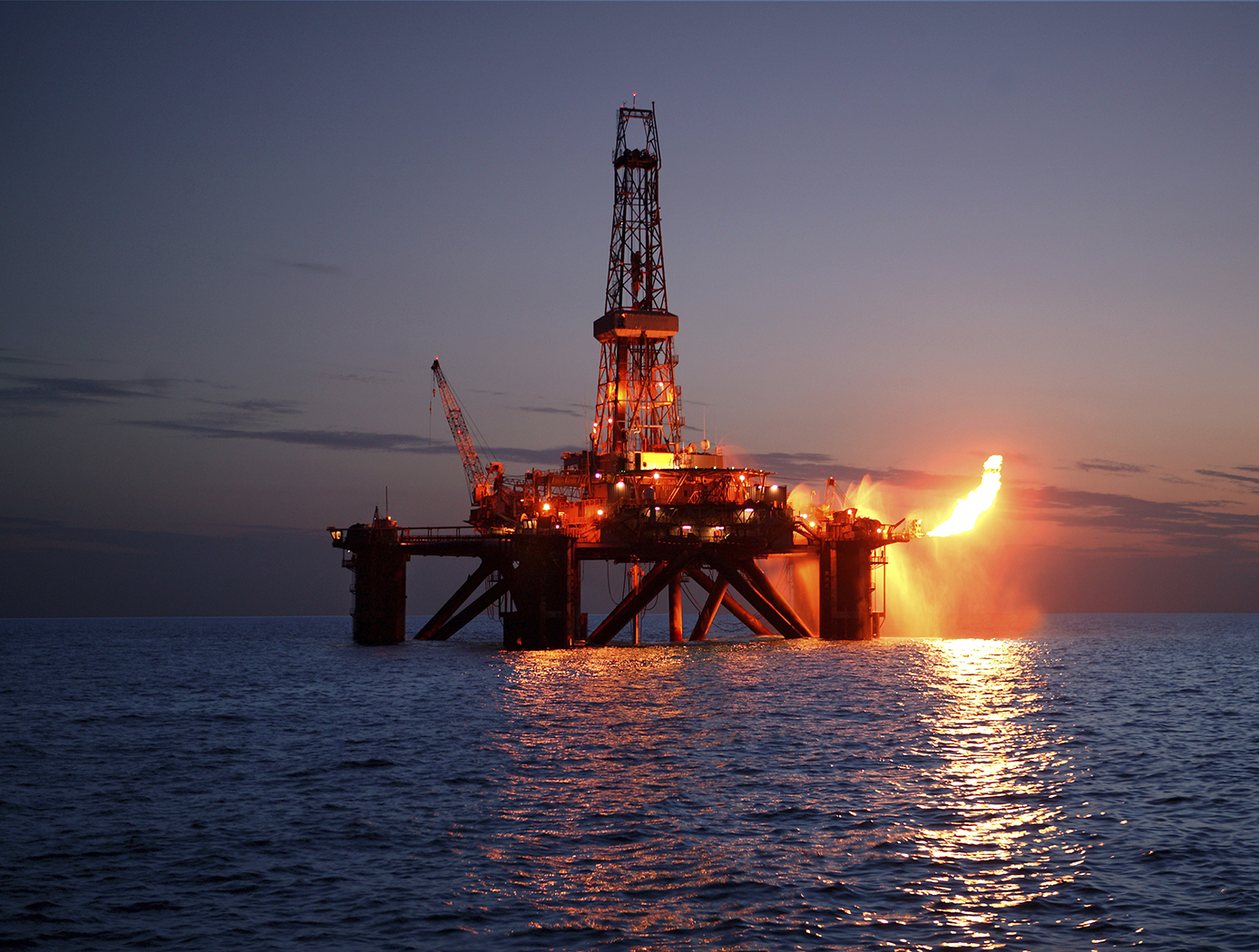
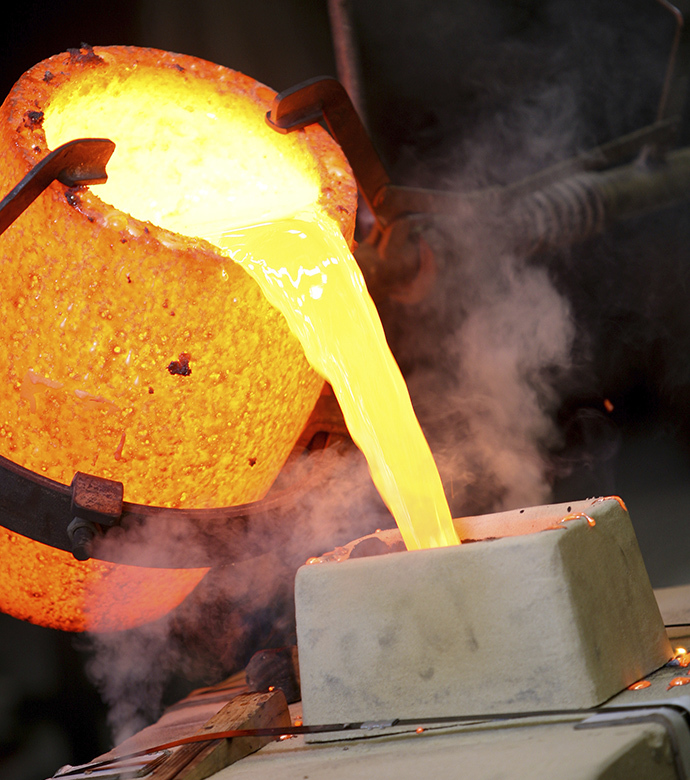
Benefits
- Versatile: Single crystal preloaders can be fabricated in a variety of geometries using different manufacturing methods
- Effective: The combination of single crystal alloys & unique preloader designs offers potential for significant advancement over current state of the art
- Robust: The single crystal preloader maintains resiliency at temperatures greater than 2000°F for short term applications
Applications
- Aerospace systems
- High-temperature manufacturing
- Industrial furnaces
- Power generation
- Oil & gas
- Chemical & material processing
Tags:
|
Similar Results

High-Temperature Ni-Based Superalloy Composition
NASA's new Ni-based superalloy uses a powder metallurgy (PM) composition that inhibits the deleterious gamma-prime to gamma-phase transformation along stacking faults during high temperature creep deformation. Ni-base superalloys have excellent high temperature properties, mostly due to the presence of coherent precipitates. At higher temperatures, these precipitates are defeated by the diffusional shear dislocations producing intrinsic and extrinsic faults. Recent studies have found that, during deformation of turbine disk alloys at high temperature, Co, Cr, and Mo segregate to these faults (removing Ni and Al) inside the strengthening precipitates of these alloys. This represents a local phase transformation from the strengthening precipitate to the weaker matrix phase. Therefore, this elemental segregation significantly weakens the ability of a precipitate to withstand further deformation, producing faster strain rates in the alloy at higher temperatures. This invention presents a solution to prevent this type of segregation along these two faults to improve the creep properties of turbine disks and similar Ni-based alloys. By alloying a specific amount of eta phase formers (Ti, Ta, Nb, and Hf), the phase transformation to can be eliminated along 2-layer extrinsic stacking faults (SESFs) in precipitates without precipitating bulk eta phase. Also, by adding a certain amount of D019 formers (Mo and W), the phase transformation to can be mitigated along 1-layer intrinsic stacking faults (SISFs) without producing bulk sigma phase. This alloy composition incorporates both strengthening methods for use in jet turbine disks, though the composition has applications in other high-stress and/or high-temperature environments as are found in power plants, space launch systems, and other critical structural applications.

Packaging for SiC Sensors and Electronics
Prior approaches to bonding a SiC sensor and a SiC cover member relied on either electrostatic bonding or direct bonding using glass frits. The problem with the former is that its relatively weak bond strength may lead to debonding during thermal cycling, while the latter requires the creation of apertures that can allow sealant to leak. Glenn's innovation uses NASA's microelectromechanical system direct chip attach (MEMS-DCA) technology that can be bulk-manufactured to reduce sensor costs. The MEMS-DCA process allows a direct connection to be made between chip and pins, thereby eliminating wire bonding. Sensors and electronics are attached in a single-stage process to a multifunctional package, which, unlike previous systems, can be directly inserted into the housing. Additional thick pins within the electrical outlet allow the package to be connected to external circuitry. Furthermore, because the top and bottom substrates' thermomechanical properties are similar to that of the sensors, the problem of mismatch in the coefficient of thermal expansion is significantly reduced, minimizing thermal cycling and component fatigue. By protecting sensors and electronics in temperatures up to 600°C, approximately twice what has previously been achievable, Glenn's innovation enables SiC components to realize one of their most exciting possibilities - direct placement within high-temperature environments.

Seal with Integrated Shroud to Protect from Exposure to Extreme Environments
Approximately 50 inches in diameter, Glenn's unique sealing system consists of multiple elements installed in a recessed rectangular sealing groove. The main sealing function is provided by an elastomer element (e.g., silicone) comprising one or more sealing wall(s) connected by a web. The wall(s) extend above the top of the sealing groove so they are compressed by the opposing mating surface during the sealing process. The retractable shroud element is installed between the wall(s), with its base resting atop the web of the sealing element. The shroud is typically composed of an elastomer material to allow for flexibility (which is essential to retraction), but it can also be made from thin metal or plastic materials. When the seal is no longer in use, a pair of V-shaped shroud "arms" extend upward from the base of the seal to cover the wall(s). A thin metal retainer is installed on top of the shroud, and fasteners pass through holes in the retainer, shroud, and sealing elements to secure the system to the base of the sealing groove. Metal washers are installed in these holes to provide a load path between the metal retainer and the surface of the sealing groove. The system can seal against either a flat metal surface or another seal of the same design. This sealing system has been designed to accommodate multiple sealing cycles and has exhibited extremely low leak rates, making it an attractive solution within a variety of industries from aerospace to agriculture.
RTV Silicone Sealing Method for Component Interfaces
NASA designed an inflatable habitat intended for space whose exterior incorporates an expandable layer known as the bladder – the main pressure shell of a module to which astronauts may reside while off-world. The bladder is made from a polymer material and is surrounded by protective layers to ensure it is not damaged and does not leak. On every module, there are two areas where the bladder and other flexible layers interface with the ends of a cylindrical core, at the bulkheads. Seals between the non-metallic bladder and the metallic bulkhead are critical in maintaining a safe pressurized environment for astronauts to live and work.
With both bulkhead plates assembled, RTV silicone is deposited in specially designed channels which are sandwiched between the plates. After the channels are filled, a cure-in-place seal is formed between the bladder and the bulkhead.
The RTV sealing method worked successfully during prototype testing as confirmed by a helium leak test and post-test visual inspection of the seals. In prototype testing, this method created a consistent and reliable seal between the bladder and bulkhead assembly replicated from the inflatable module design. The RTV sealing method may benefit terrestrial applications that may demand cure-in-place internal seals. The method could also innovate manufacturing processes for components by enhancing the speed of assembly while increasing seal integrity.

Woven Thermal Protection System
Going farther, faster and hotter in space means innovating how NASA constructs the materials used for heat shields. For HEEET, this results in the use of dual-layer, three-dimensional, woven materials capable of reducing entry loads and lowering the mass of heat shields by up to 40%. The outer layer, exposed to a harsh environment during atmospheric entry, consists of a fine, dense weave using carbon yarns. The inner layer is a low-density, thermally insulating weave consisting of a special yarn that blends together carbon and flame-resistant phenolic materials. Heat shield designers can adjust the thickness of the inner layer to keep temperatures low enough to protect against the extreme heat of entering an atmosphere, allowing the heat shield to be bonded onto the structure of the spacecraft itself. The outer and inner layers are woven together in three dimensions, mechanically interlocking them so they cannot come apart. To create this material, manufacturers employ a 3-D weaving process that is similar to that used to weave a 2-D cloth or a rug. For HEEET, computer-controlled looms precisely place the yarns to make this kind of complex three-dimensional weave possible. The materials are woven into flat panels that are formed to fit the shape of the capsule forebody. Then the panels are infused with a low-density version of phenolic material that holds the yarns together and fills the space between them in the weave, resulting in a sturdy final structure. As the size of each finished piece of HEEET material is limited by the size of the loom used to weave the material, the HEEET heat shield is made out of a series of tiles. At the points where each tile connects, the gaps are filled through inventive designs to bond the tiles together.