Robotic System for Infra-structure Reconnaissance
Robotics Automation and Control
Robotic System for Infra-structure Reconnaissance (MSC-TOPS-70)
Facilitates remote video and sonar investigation of piping networks
Overview
Innovators at NASA Johnson Space Center have developed a robotic system whose primary structural platform, or “orb”, can be injected into a pipe network and perform reconnaissance of piping infrastructure and other interior volumes. When deployed, this technology uses throttled fluid flow from a companion device for passive propulsion. A tethered line facilitates directional control by the orb’s operator, allowing it to navigate through various piping configurations, including 90-degree junctions.
Inspired by NASA’s Robonaut program for space applications, this technology was developed to facilitate a cost-effective method to inspect deep underwater oil wells residing under oceanic drilling platforms slated for decommission. Instead of initiating an expensive and environmentally risky operation to perforate the containment system of an underwater oil well to inspect its contents, the orb can be navigated through existing pipe networks to conduct real-time video and sonar investigations.
The modular orb design accepts a variety of plug-and-play sensors along with a potential sample retrieving device, making it adaptable to different environments. Its housing can be easily disassembled in the field, enabl-ing a change or maintenance of its sensors or electronics. Because piping exists in a myriad of industries, such as in refineries, manufacturing and power plants, sewage systems, etc., plant operators could need a cost-effective reconnaissance device of this nature.
The Technology
The robotic system is comprised of six main components: the orb that performs the reconnaissance, an orb injector housing that attaches to a piping network, a tether and reel subsystem that attaches to the back of the injector housing, a fluid injection subsystem that attaches toward the front of the injector housing, an external power and data subsystem, and associated control and monitoring software.
Usage of the system begins with an operator attaching the injector housing, with the orb stowed inside, to a flanged gate valve belonging to the piping network of concern. Requisite power, data, and fluid subsystems are attached, and the system is energized for usage. The orb is released via the tether and reel, and a controlled fluid force is imparted on the orb to help guide it along its mission. The tether supplies power and guidance to the orb, and relays real-time data back to the operator.
The orb’s interior features a modular plug-and-play architecture which may comprise COTS instrumentation for reconnaissance or investiga-tion, LIDAR, and inertial measuring and motion sensors. This instru-mentation could be used in combination with other sub-systems such as lighting, and core and sample retrieving mechanisms. These com-ponents are supported by other onboard devices such as a CPU, power source and controller, and data transmission encoders and multiplexers.
The Robotic System for Infrastructure Reconnaissance is at TRL 8 (actual system completed and "flight qualified" through test and demonstration), and is now available for licensing. Please note that NASA does not manufacture products itself for commercial sale.
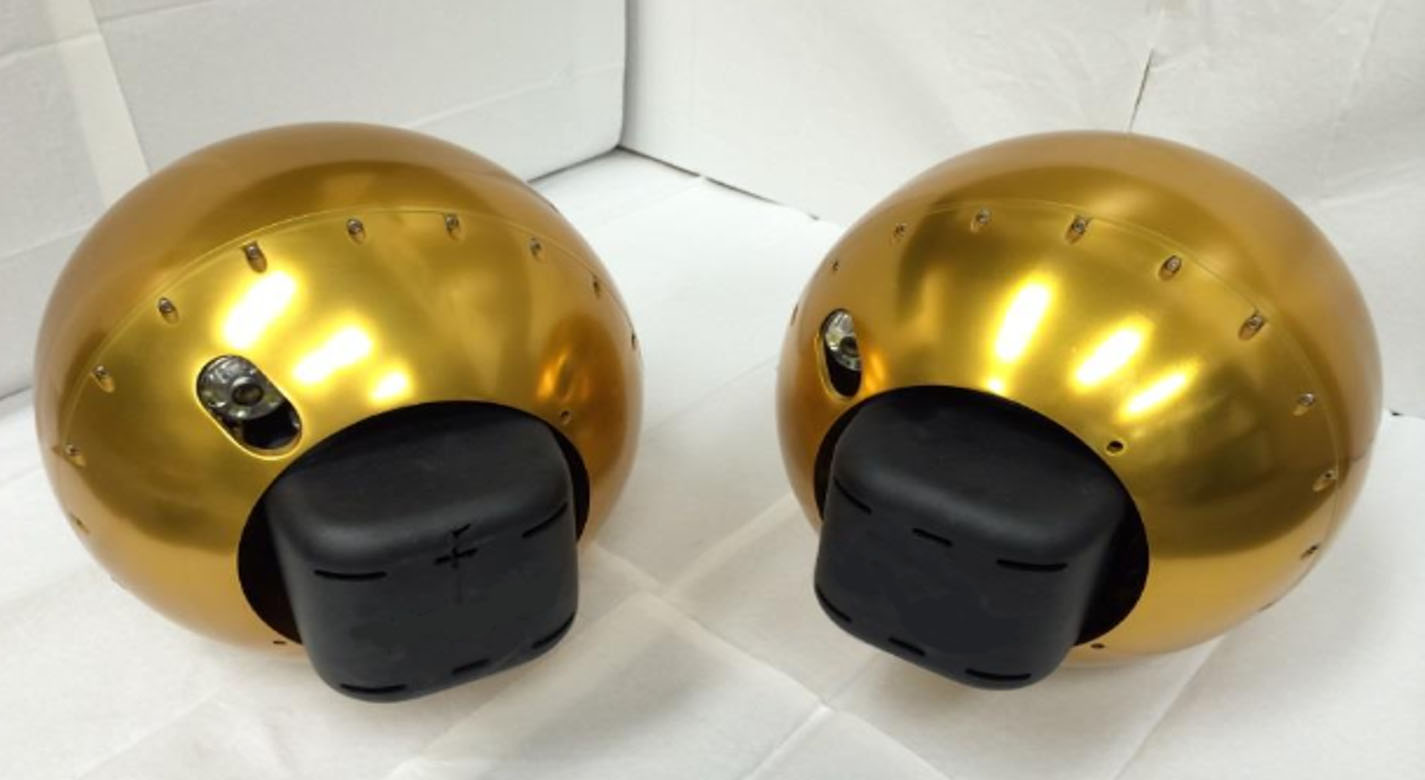
Benefits
- Facilitates real-time investigation of industrial pipe and well networks
- Provides real-time camera and sonar data to operator(s)
- Features modular architecture for hardware customization
- Reconfigurable for different environments
- Allows easy disassembly for field servicing
- Agile inspection orb navigates 90-degree pipe junctions
- System comprises control and monitoring software
- Inexpensive to operate
- Comprises COTS components
- Reduces environmental risk for subsea well inspection
- Proven performance
Applications
- Oil and gas industries
- Industrial refineries
- Energy production infrastructures
- Manufacturing plants
- Any pipeline and connected structure allowing for fluid flow
Similar Results

Regolith Advanced Surface Systems Operations Robot (RASSOR) Excavator
Regolith excavation is desired in future space missions for the purpose of In Situ Resource Utilization (ISRU) to make local commodities, such as propellants and breathing air, and to pursue construction operations. The excavation of regolith on another planetary body surface, such as the Moon, Mars, an asteroid, or a comet is extremely difficult because of the high bulk density of regolith at lower depths. Additionally, because of the low gravity in these space surface environments, the mass of the excavator vehicle does not provide enough reaction force to enable the excavation blade to penetrate the regolith if traditional terrestrial methods are used.
RASSOR uses counterrotating bucket drums on opposing arms to provide near-zero horizontal and minimal vertical net reaction force so that excavation is not reliant on the traction or weight of the mobility system to provide a reaction force to counteract the excavation force in low-gravity environments. The excavator can traverse steep slopes and rough terrain, and its symmetrical design enables it to operate in reverse so that it can recover from overturning by continuing to dig in the new orientation. The system is capable of standing up in a vertical position to dump into a receiving hopper without using a ramp. This eliminates the need for an onboard dump bin, thus reducing complexity and weight.
During loading, the bucket drums excavate soil/regolith by scoops mounted on the drums exteriors that sequentially take multiple cuts of soil/regolith while rotating at approximately 20 revolutions per minute. During hauling, the bucket drums are raised by rotating the arms to provide clearance above the surface being excavated. The mobility platform can then travel while the soil/regolith remains in the raised bucket drums. When the excavator reaches the dump location, the bucket drums are commanded to reverse their direction of rotation, which causes soil/regolith to be expelled out of each successive scoop. RASSOR has wireless control, telemetry, and onboard transmitting cameras, allowing for teleoperation with situational awareness. The unit can be programmed to operate autonomously for selected tasks.

Robotic gripper for satellite capture and servicing
The Gripper is located at the end of a robotic system consisting of a robotic arm equipped with a Tool Drive or End Effector comprising the input actuator to the Gripper as well as the structural, power and data link between the Gripper and the robotic arm. In a notional concept of operations, a Servicer would approach the Client in an autonomous rendezvous and capture (AR&C) maneuver. When the Servicers sensor suite confirms that the distance, orientation, and relative translational and angular rates with respect to the Client are within an acceptable range, the Servicer enables the grasping sequence, where the robotic arm, equipped with Gripper, extend forward to the Client. When the Gripper/ Servicer sensors indicate that the Client marman ring is sufficiently within the capture range of the Gripper, a trigger signal is sent to the robot control system that commands the End Effector to drive the mechanism of the Gripper and affect closure around the marman ring. The Gripper consists of a pair of jaws which are driven by an internal transmission. The transmission receives input torque from the End Effector and converts the torque to appropriate motion of the jaws.

Robot-Driven Blind Mate Interface
The Robot-Driven Blind Mate Interface is a specialized interface utilizing a robot-driven, blind mate mechanism that allows structural, electrical, and fluid connections to be reliably made in a single motion. The interface is composed of a removable side and a fixed side. The removable side consists of the robot grasp point, a drive bolt, one side of a blind mate fluid, electrical couplings, and one side of the interface alignment features. The fixed side consists of corresponding alignment features and the mechanisms carriage. The carriage houses the corresponding fluid and electrical couplings and over-travel protection for the couplings. The robot system used dictates the specific type of robot grasp point, any required targets, and mechanism status indicators.
The mate and de-mate forces of the interface are balanced throughout the mechanism so it can be actuated with one motion, such as turning a single drive bolt. The point at which the different connectors seat is carefully controlled the spring forces distributed throughout the mechanism. For example, the electrical connectors can make contact before the fluid couplings, if desirable, to accommodate the long length of high voltage and current pins. The springs that compress to provide over-travel protection on the electrical connectors allow for preload to be developed between the removable side and fixed side of the interface to create a sound structural connection while not over-stressing the connectors. Overtravel protection can be applied to fluid couplings as needed depending on the specific coupling used. The interface is versatile and can be tailored to a wide range of fluid and electrical couplings.

Fiber Optic Sensing Technologies
The FOSS technology revolutionizes fiber optic sensing by using its innovative algorithms to calculate a range of useful parameters—any and all of which can be monitored simultaneously and in real time. FOSS also couples these cutting-edge algorithms with a high-speed, low-cost processing platform and interrogator to create a single, robust, stand-alone instrumentation system. The system distributes thousands of sensors in a vast network—much like the human body's nervous system—that provides valuable information.
How It Works
Fiber Bragg grating (FBG) sensors are embedded in an optical fiber at intervals as small as 0.25 inches, which is then attached to or integrated into the structure. An innovative, low-cost, temperature-tuned distributed feedback (DFB) laser with no moving parts interrogates the FBG sensors as they respond to changes in optical wavelength resulting from stress or pressure on the structure, sending the data to a processing system. Unique algorithms correlate optical response to displacement data, calculating the shape and movement of the optical fiber (and, by extension, the structure) in real time, without affecting the structure's intrinsic properties. The system uses these data to calculate additional parameters, displaying parameters such as 2D and 3D shape/position, temperature, liquid level, stiffness, strength, pressure, stress, and operational loads.
Why It Is Better
FOSS monitors strain, stresses, structural instabilities, temperature distributions, and a plethora of other engineering measurements in real time with a single instrumentation system weighing less than 10 pounds. FOSS can also discern between liquid and gas states in a tank or other container, providing accurate measurements at 0.25-inch intervals. Adaptive spatial resolution features enable faster signal processing and precision measurement only when and where it is needed, saving time and resources. As a result, FOSS lends itself well to long-term bandwidth-limited monitoring of structures that experience few variations but could be vulnerable as anomalies occur (e.g., a bridge stressed by strong wind gusts or an earthquake).
As a single example of the value FOSS can provide, consider oil and gas drilling applications. The FOSS technology could be incorporated into specialized drill heads to sense drill direction as well as temperature and pressure. Because FOSS accurately determines the drill shape, users can position the drill head exactly as needed. Temperature and pressure indicate the health of the drill. This type of strain and temperature monitoring could also be applied to sophisticated industrial bore scope usage in drilling and exploration.
For more information about the full portfolio of FOSS technologies, see visit https://technology-afrc.ndc.nasa.gov/featurestory/fiber-optic-sensing

Lunar Surface Manipulation System
NASA Langley developed the LSMS because of the need for a versatile system capable of performing multiple functions on the lunar surface, such as unloading components from a lander, transporting components to an operational site and installing them, and supporting service and replacement during component life. Current devices used for in-space operations are designed to work on orbit (zero g) only and thus do not have sufficient strength to operate on planetary surfaces. Traditional cranes are specialized to the task of lifting and are not capable of manipulator-type positioning operations.
The innovations incorporated into the LSMS allow it to lower payloads to the ground over a significant portion of the workspace without use of a hoist, functioning like a robot manipulator, thus providing a rigid connection and very precise control of the payload. The LSMS uses a truss architecture with pure compression and tension members to achieve a lightweight design. The innovation of using multiple spreaders (like spokes in a wheel) allows the LSMS to maintain its high structural efficiency throughout its full range of motion. Rod portions of the tension members automatically lift off and re-engage the spreaders as the joint articulates, allowing a large range of motion while maintaining mechanical advantage. In addition, the LSMS uses a quick-change device at the tip end that enables automated acquisition of end effectors or special purpose tools to increase its versatility.