3D Mineralized Bone Constructs
Health Medicine and Biotechnology
3D Mineralized Bone Constructs (MSC-TOPS-95)
Human bone-like structures grown in a lab can eliminate in vivo testing
Overview
Innovators at NASA Johnson Space Center have developed a technique to grow 3D tissue constructs, similar to human bone, in a laboratory environment. Problems arise when studying both the normal state and pathophysiology of bone. As an organ system, it is slow growing, so the time to study and observe a response to a particular stimulus is relatively long. Our bioengineers have discovered that osteoblast and osteoclast cell types can be induced to aggregate into large spheroids in a specific spatial relationship under certain culture conditions when placed in a rotating-wall tissue-culture vessel (shown above). The ability to construct a 3D model of such mineralized tissue on-demand, using a co-culture of human cells that differentiate and spatially arrange themselves in a physiologically relevant manner, is a major step forward in how the process of bone formation and remodeling can be studied.
The Technology
One of the central objectives of this project was the development and characterization of a 3D mineralized tissue model system in which the effects of mechanical load (e.g., compression loading, tension, vibration, etc.) on the cellular responses of osteoblasts and osteoclasts could be investigated. After introducing mineralization agents to the culture, the constructs take on a bone-like appearance and have a more rigid structure suitable for being tested. Testing of the mineralized constructs confirmed the presence of calcium through a crystalline matrix histochemical stain. The central core is void of necrotic material, instead filled by a crystalline matrix with embedded nucleated cells. Remarkably, the nucleated cells do not express osteoblast markers, indicating differentiation to the in vivo cell type known as the osteocyte. In addition, as is characteristic to native periosteum, osteoclast precursor cells were imaged and proven to naturally arrange as an outer layer of the mineralized bone tissue construct. Development of this model will provide a unique venue for testing proposed countermeasures to space flight-induced bone loss. It will also allow a mechanistic approach in the modulation of cell signaling at the cellular level within the bone matrix.
The Development And Characterization Of A Three-Dimensional Tissue Culture Model Of Bone is a technology readiness level (TRL) 6 (system/subsystem prototype demonstrated in a relevant environment). The innovation is now available for your company to license. Please note that NASA does not manufacture products itself for commercial sale.
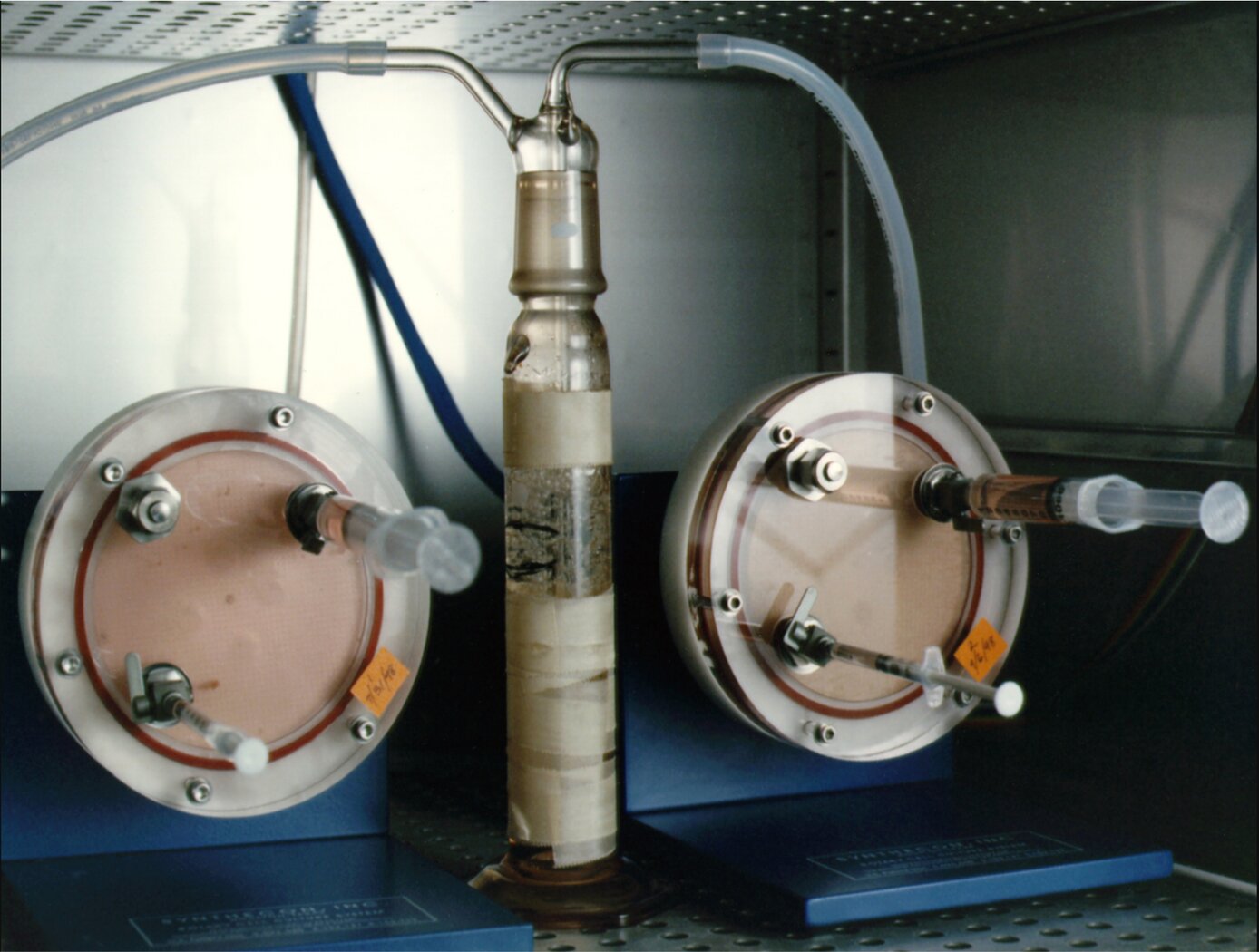
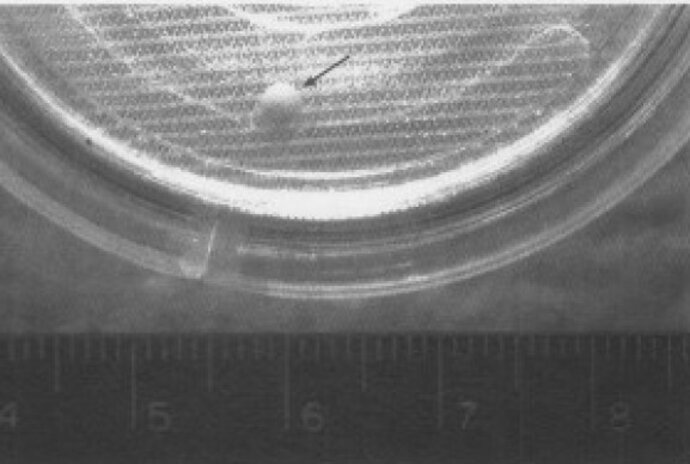
Benefits
- Builds better representation of in vivo bone formation processes compared to traditional osteoblast only models
- Low-cost alternative for studying the process of bone loss and formation
- Rapid on-demand 3D model construction
- Provides a model for studying both normal bone physiology and pathological responses observed in disease states
- High economic platform for drug discovery
- Allows for easier study of interface between bone and prosthetic devices
Applications
- Medical Devices: orthopedic research and product development
- Pharmaceuticals: drug discovery
- Research: bone function and formation studies; bone physiology and pathological responses to environmental influence and insults such as radiation
Tags:
|
Similar Results

Human Tissue-Like Cellular Assemblies Grown for Respiratory Studies
In vitro three-dimensional (3D) human broncho-epithelial (HBE) tissue-like assemblies (3D HBE TLAs or TLAs) were engineered in modeled microgravity using rotating wall vessel technology (pictured above) to mimic the characteristics of in vivo tissue. The TLAs were bioengineered onto collagen-coated cyclodextran beads using primary human mesenchymal bronchial-tracheal cells (HBTC) as the foundation matrix and an adult human broncho-epithelial immortalized cell line (BEAS-2B) as the overlying component. The resulting TLAs share significant characteristics with in vivo human respiratory epithelium including polarization, tight junctions, desmosomes, and microvilli. The presence of tissue-like differentiation markers including villi, keratins, and specific lung epithelium markers, as well as the production of tissue mucin, further confirm these TLAs have differentiated into tissues functionally like in vivo tissues. TLAs mimic aspects of the human respiratory epithelium and provide a unique capability to study the interactions of respiratory viruses and their primary target tissue independent of the host's immune system.
The innovation "Methods For Growing Tissue-Like 3D Assemblies Of Human Broncho-Epithelial Cells" is at Technology Readiness Level (TRL) 6 (which means system/subsystem prototype demonstration in a relevant environment) and the related patent is now available to license for development into a commercial product. Please note that NASA does not manufacture products itself for commercial sale.

Ionic Magnetic Resonance Tailors Animal Cells/Tissues
The apparatus comprises a randomized gravity vector multiphasic culture system with a self-feeding growth module, an optionally disposable nutrient module, and a removable AIMR chamber that delivers a pulsating multivariant field to the contents of the culture system. It produces overlapping or fluctuating alternating ionic magnetic resonance frequencies at one or more modal intervals ranging from about 7.8 Hz to about 59.9 Hz to the cell chamber. The apparatus may yield better regulation that can be manipulated to allow for increased rate of cell growth, faster differentiation, increased cell fidelity, and the induction or suppression of selective physiological genes involved in directing cellular differentiation and dedifferentiation.
The use of an AIMR field may provide a significant improvement over existing bioreactors, including pulsating electromagnetic field (PEMF) and time-variance electromagnetic field (TVEMF) cellular growth induced systems, in that AIMR incorporates the modulation of cellular transcription. The AIMR system utilizes pre-sterilized disposable modules and a removable alternating ionic magnetic resonance chamber, reducing the hazard for contamination, allowing scientists to implement physiological and homeostatic parameters similar to a naturally occurring physiological system.

Electroactive Scaffold
Current scaffold designs and materials do not provide all of the appropriate cues necessary to mimic in-vivo conditions for tissue engineering and stem cell engineering applications. It has been hypothesized that many biomaterials, such as bone, muscle, brain and heart tissue exhibit piezoelectric and ferroelectric properties. Typical cell seeding environments incorporate biochemical cues and more recently mechanical stimuli, however, electrical cues have just recently been incorporated in standard in-vitro examinations. In order to develop their potential further, novel scaffolds are required to provide adequate cues in the in-vitro environment to direct stem cells to differentiate down controlled pathways or develop novel tissue constructs. This invention is for a scaffold that provides for such cues by mimicking the native biological environment, including biochemical, topographical, mechanical and electrical cues.

Micro-Organ Device Mimics Organ Structures for Lab Testing
The MOD platform technology represents a small, lightweight, and reproducible in vitro drug screening model that could inexpensively mimic different mammalian tissues for a multitude of applications. The technology is automated and imposes minimal demands for resources (power, analytes, and fluids). The MOD technology uses titanium isopropoxide to bond a microscale support to a substrate and uses biopatterning and 3D tissue bioprinting on a microfluidic microchip to eliminate variations in local seeding density while minimizing selection pressure. With the MOD, pharmaceutical companies can test more candidates and concentrate on those with more promise therefore, reducing R&D overall cost.
This innovation overcomes major disadvantages of conventional in vitro and in vivo experimentation for purposes of investigating effects of medicines, toxins, and possibly other foreign substances. For example, the MOD platform technology could host life-like miniature assemblies of human cells and the effects observed in tests performed could potentially be extrapolated more readily to humans than could effects observed in conventional in vivo cell cultures, making it possible to reduce or eliminate experimentation on animals.
The automated NASA developed technology with minimal footprint and power requirements, micro-volumes of fluids and waste, high throughput and parallel analyses on the same chip, could advance the research and development for new drugs and materials.

3D Construction of Biologically Derived Materials
Once genes for a desired material type, delivery mode, control method and affinity have been chosen, assembling the genetic components and creating the cell lines can be done with well-established synthetic biology techniques. A 3D microdeposition system is used to make a 3D array of these cells in a precise, microstructure pattern and shape.
The engineered cells are suspended in a printable 'ink'. The 3D microdeposition system deposits minute droplets of the cells onto a substrates surface in a designed print pattern. Additional printer passes thicken the material. The cell array is fed nutrients and reagents to activate the engineered genes within the cells to create and deposit the desired molecules. These molecules form the designed new material. If desired, the cells may be removed by flushing. The end product is thus a 3D composite microstructure comprising the novel material.
This innovation provides a fast, controlled production of natural, synthetic, and novel biomaterials with minimum resource overhead and reduced pre- and post-processing requirements.