Transformable Hypersonic Aerodynamic Decelerator
aerospace
Transformable Hypersonic Aerodynamic Decelerator (TOP2-162)
Transformable and Reconfigurable Entry, Descent and Landing Systems and Methods
Overview
NASA has developed a game changing deployable aeroshell concept for entry, descent and landing (EDL) of large science and exploration-class payloads.The Adaptable, Deployable Entry Placement Technology (ADEPT) concept is a mechanically deployable semi-rigid aeroshell entry system capable of achieving low ballistic coefficient during entry suitable for a variety of planetary or earth return missions. It leverages Ames expertise in Thermal Protection systems (TPS) material and entry system design, development and testing. The deployable decelerator systems offer a lighter-weight solution to current rigid, high ballistic coefficient aeroshells. The deployable feature of ADEPT allows each mission to utilize an entry system design that fits within existing launch vehicle systems and later transforms into a low ballistic coefficient configuration for EDL. Consisting of rigid ribs and a TPS, deployment can be done for inspection in Earth orbit by extending the ribs and stretching the TPS in between (in a method similar to an opening umbrella) and thereby reducing the mission risk.
The Technology
The invention allows the deployment of a large aerodynamic decelerator relative to the size of its launch vehicle, which is controllable and can be transformed into a landing system. A structure composed of a radial assembly of ribs and struts in a four bar linkage arrangement fits inside a launch vehicle shroud, expands into a deployed size, and permits rotation about a pivot point along the vehicle axis. The mechanism that deploys the decelerator surface, doubles as the actuation/control mechanism, and triples as the payload surface leveling system. The design permits the use of conformable thermal protection systems at the central part and a flexible TPS, 3-D woven carbon fabric, as skin in the majority of the regions of the aeroshell entry system. The fabric handles both the heat and mechanical load generated during entry. This system is very mass competitive with other lightweight systems such as inflatable and rigid decelerators and is believed to be more reliable and testable at sub-scale. Once the payload reaches its destination, the decelerator structure leverages atmospheric drag to slow the craft from hypersonic travel speeds to an appropriate landing velocity. The decelerator can be actuated during descent to generate lift and steer the payload to its intended destination. Retro propulsion engines provide the final deceleration just before landing, and the decelerator structure is inverted to act as a landing platform and help minimize the impact of landing load.
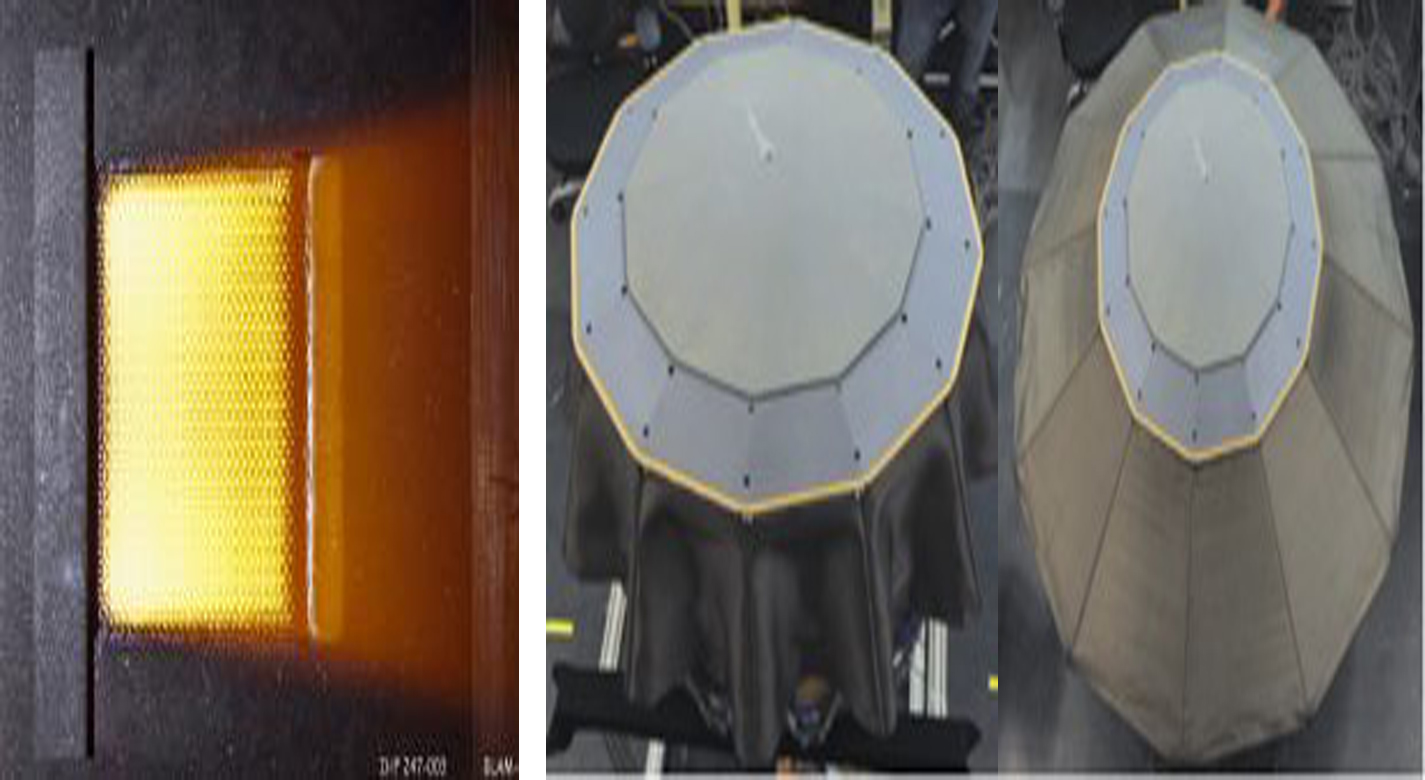
Benefits
- Light weight
- Easily deployable
- Aerodynamic surface can be actuated
- Design can be scaled to fit any size craft or payload
- Support packaging within the launch shroud
- Facilitate redirection
- Accommodate a retro-propulsion system
- Offers a compact entry system solution during the EDL mission segment.
- A single ADEPT can perform both aerocapture and atmospheric EDL.
Applications
- Human and heavy payload Mars missions
- Robotic missions to Venus and Mars
- Small satellite retrieval missions
Similar Results

Woven Thermal Protection System
Going farther, faster and hotter in space means innovating how NASA constructs the materials used for heat shields. For HEEET, this results in the use of dual-layer, three-dimensional, woven materials capable of reducing entry loads and lowering the mass of heat shields by up to 40%. The outer layer, exposed to a harsh environment during atmospheric entry, consists of a fine, dense weave using carbon yarns. The inner layer is a low-density, thermally insulating weave consisting of a special yarn that blends together carbon and flame-resistant phenolic materials. Heat shield designers can adjust the thickness of the inner layer to keep temperatures low enough to protect against the extreme heat of entering an atmosphere, allowing the heat shield to be bonded onto the structure of the spacecraft itself. The outer and inner layers are woven together in three dimensions, mechanically interlocking them so they cannot come apart. To create this material, manufacturers employ a 3-D weaving process that is similar to that used to weave a 2-D cloth or a rug. For HEEET, computer-controlled looms precisely place the yarns to make this kind of complex three-dimensional weave possible. The materials are woven into flat panels that are formed to fit the shape of the capsule forebody. Then the panels are infused with a low-density version of phenolic material that holds the yarns together and fills the space between them in the weave, resulting in a sturdy final structure. As the size of each finished piece of HEEET material is limited by the size of the loom used to weave the material, the HEEET heat shield is made out of a series of tiles. At the points where each tile connects, the gaps are filled through inventive designs to bond the tiles together.

Aerodynamically Actuated Thrust Vectoring Device
The thrust actuating device includes several innovations in the aerodynamically stable tilt actuation of propellers, propeller pylons, jets, wings, and fuselages, collectively called propulsors. The propulsors rotate between hover and forward flight mode for a tilt-wing or tilt-rotor aircraft. A vehicle designed using this technology can transition from a hovering flight condition to a wing born flight condition with no mechanical actuation and can do so without complex control systems. This results in a reduction in system weight and complexity and produces a robust and naturally stable hovering aircraft with efficient forward flight modes.

Thermal protection supplement for reducing interface thermal mismatch
The invention includes an exposed surface cap with a specially formulated coating, an insulator base adjacent to the cap with another specially formulated coating, and one or more pins that extend from the cap through the insulator base to tie the cap and base together through ceramic bonding and mechanical attachment. The cap and insulator base have corresponding depressions and projections that mate and allow for differences in thermal expansion of the cap and base. The cap includes a high-temperature, low density, carbonaceous, fibrous material whose surface is optionally treated with a High Efficiency Tantalum-based Ceramic Composite (HETC) formulation, the fibrous material being drawn from the group consisting of silicon carbide foam and similar porous, high temperature materials. The insulator base and pin(s) contain similar material. The mechanical design is arranged so that thermal expansion differences in the component materials (e.g., cap and insulator base) are easily tolerated. It is applicable to both sharp and blunt leading edge vehicles. This extends the possible application of fibrous insulation to the wing leading edge and/or nose cap on a hypersonic vehicle. The lightweight system comprises a treated carbonaceous cap composed of Refractory Oxidation-resistant Ceramic Carbon Insulation (ROCCI), which provides dimensional stability to the outer mold line, while the fibrous base material provides maximum thermal insulation for the vehicle structure. The composite has graded surface treatments applied by impregnation to both the cap and base. These treatments enable it to survive in an aero-convectively heated environment of high-speed planetary entry. The exact cap and base materials are chosen in combination with modified surface treatments and a specially formulated surface coating, taking into account the duration of exposure and expected surface temperatures for the particular application.

Fixed Wing Angle eVTOL
While previous eVTOLs often require a near 90° wing tilt to position propellers in an optimal location to generate vertical force for takeoff, NASA has taken a very different approach. NASA's design instead uses a slight wing angle and large flaps designed to deflect slipstream generated by the propellers to create a net positive force in the vertical direction, all while preventing forward movement. This unique configuration allows for takeoff and landing operations without the need for near 90° wing tilt angles. After takeoff, the transition to forward flight only requires a slight change in attitude of the vehicle and retraction of the flaps. Similar solutions require large changes in attitude to accomplish this transition which is often undesirable, especially for air taxi operations that involve passengers.
Given the effectiveness of this configuration for generating upward force, the requirement for wing angle tilt has been reduced from near 90° to approximately 15° during takeoff. Further iterations may reduce this requirement even further to 0°. By eliminating the need for near 90° wing tilt, NASA's eVTOL design removes the need for mechanisms to perform active tilting of the wings or rotors, reducing system mass and thereby improving performance. Flaps represent the only components that require actuation for takeoff and landing operations.
Innovators at NASA leveraged the Langley Aerodrome 8 (LA-8), a modular testbed vehicle that allows for rapid prototyping and testing of eVTOLs with various configurations, to design and test this novel concept.

Aerospace Vehicle Entry Flightpath Control
This novel flightpath control system exploits the dihedral effect to control the bank angle of the vehicle by modulating sideslip (Figure 1). Exploiting the dihedral effect, in combination with significant aerodynamic forces, enables faster bank accelerations than could be practically achieved through typical control strategies, enhancing vehicle maneuverability. This approach enables vehicle designs with fewer control actuators since roll-specific actuators are not required to regulate bank angle. The proposed control method has been studied with three actuator systems (figure below), Flaps Control System (FCS); Mass Movement Control System (MMCS); and Reaction Control System (RCS).
• FCS consists of a flap configuration with longitudinal flaps for independent pitch control, and lateral flaps generating yaw moments. The flaps are mounted to the shoulder of the vehicle’s deployable rib structure. Additionally, the flaps are commanded and controlled to rotate into or out of the flow. This creates changes in the vehicle’s aerodynamics to maneuver the vehicle without the use of thrusters.
• MMCS consists of moveable masses that are mounted to several ribs of the DEV heatshield, steering the vehicle by shifting the vehicle’s Center of Mass (CoM). Shifting the vehicle’s CoM adjusts the moment arms of the forces on the vehicle and changes the pitch and yaw moments to control the vehicle’s flightpath.
• RCS thrusters are mounted to four ribs of the open-back DEV heatshield structure to provide efficient bank angle control of the vehicle by changing the vehicle’s roll. Combining rib-mounted RCS thrusters with a Deployable Entry Vehicle (DEV) is expected to provide greater downmass capability than a rigid capsule sized for the same launch