Method for Transferring a Spacecraft from Geosynchronous Transfer Orbit to Lunar Orbit
aerospace
Method for Transferring a Spacecraft from Geosynchronous Transfer Orbit to Lunar Orbit (TOP2-272)
Spacecraft rideshare via a GEO launch
Overview
This novel innovation from Ames Research Center allows spacecraft to share rides with larger spacecraft which are headed to Geosynchronous Earth Orbit (GEO). The secondary spacecraft is dropped off Geosynchronous Transfer Orbit (GTO) at any time during the day or year and will subsequently enter lunar orbit, with no constraint on the lunar orbit inclination. The secondary spacecraft can be relatively small, riding as a secondary payload with a larger primary spacecraft. The secondary spacecraft is intended to be controllable (i.e., maneuverable).
The Technology
The invention presents a trajectory design whereby a spacecraft can be launched as a secondary payload into a Geosynchronous Transfer Orbit (GTO) and through a series of maneuvers to reach lunar orbit. The trajectory analysis begins by identifying acceptable ranges of lunar orbit altitude and inclination values. The unique features of this method includes the use of either a leading or trailing edge lunar flyby to achieve an orbit inclination in the lunar orbit plane from a GTO launched at any time of day. This technique is applicable to secondary spacecraft that share a ride to space resulting in a substantially reduced cost, and with no control of the launch conditions. Major advantages of this design include the relatively short (maximum) lunar transfer duration (<3 months, less than half of that required for a Sun-Earth weak-stability boundary transfer), simplicity and consistency of design (again compared to a Sun-Earth weak stability boundary transfer).
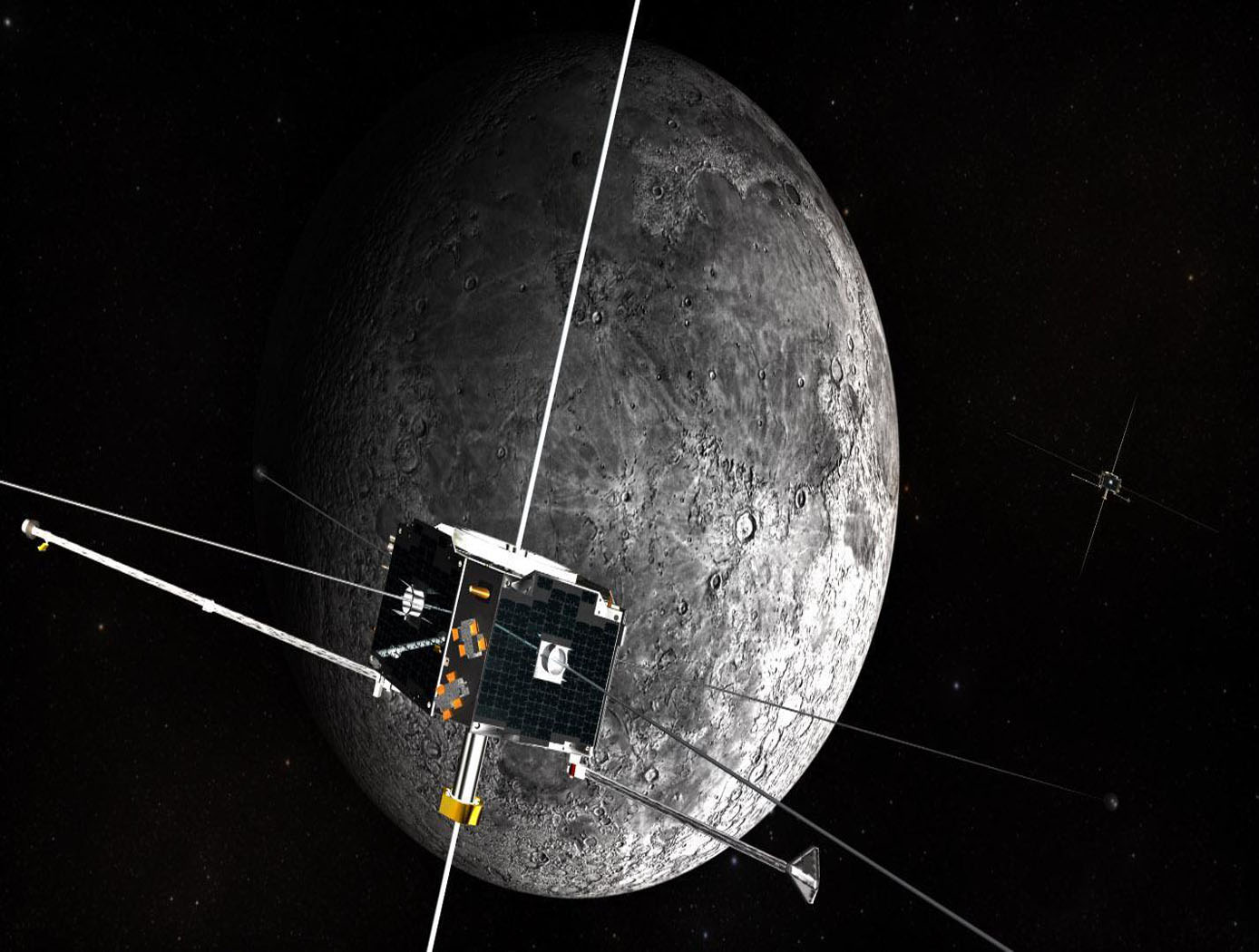
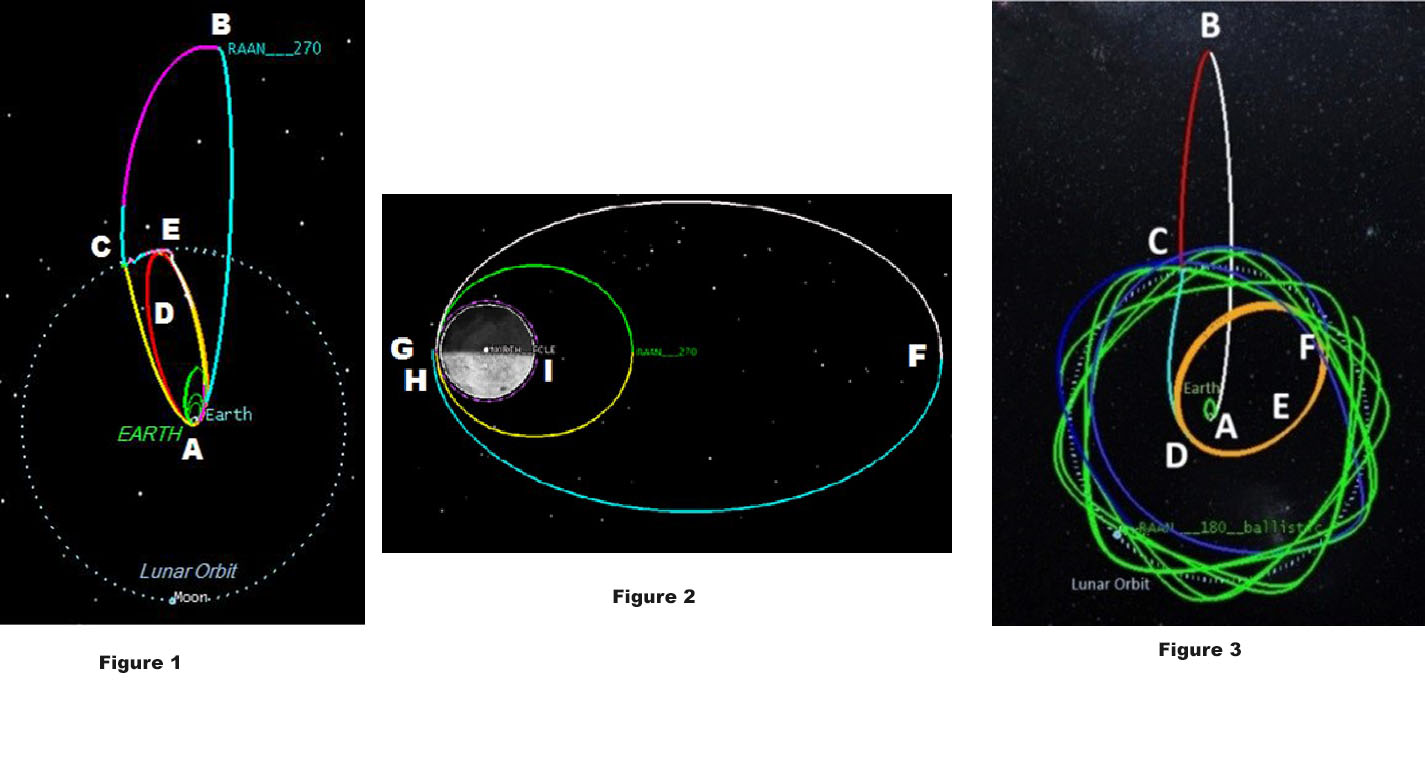
Benefits
- A relatively small and maneuverable spacecraft can ride as a secondary payload with a larger primary spacecraft decreasing launch cost
- Enter lunar orbit with possibility of achieving any inclination and perilune altitude from an initial GTO
- There are no restrictions on launch time or date
- Increased launch flexibility
- Shorter mission duration
- Consistency of design
Applications
- Moon Exploration
- Establishing lunar orbit
- Moon observation
- Mining of Helium-3 nuclear fuel
- Launch-base for space missions
- Mining of rare earth elements
- Geology
- Mapping
- Astronomy
- Space communication
Technology Details
aerospace
TOP2-272
ARC-17743-1
- Genova, A. L. et. al, Trajectory Design from GTO to Lunar Equatorial Orbit for the Dark Ages Radio Explorer Spacecraft, Proceedings of the 25th AAS/AIAA Space Flight Mech. Mtg., Williamsburg, VA, Jan. 11-15, 2015.
- Genova, A. L., & Kaplinger, B., From GTO to Ballistic Lunar Capture using an Interior Lagrange Point Transfer, Proceedings of the 27th AAS/AIAA Astrodynamics Specialist Conf., Stevenson, WA 98648, Aug. 20-24, 2017.
- Genova, A. L., & Kaplinger, B., From GTO to Ballistic Lunar Capture using an Interior Lagrange Point Transfer, Proceedings of the 27th AAS/AIAA Astrodynamics Specialist Conf., Stevenson, WA 98648, Aug. 20-24, 2017.
Similar Results

The Navigator GPS Receiver
To enable it to acquire GPS signals very quickly and also track weak signals, the radiation-hardened Navigator receiver utilizes a bank of hardware correlators, a ColdFire microprocessor, and a specialized fast acquisition module (see figure 1). The hardware is implemented in VHSIC Hardware Description Language (VHDL) to target radiation-hardened Field Programmable Gate Arrays (FPGA) rather than Application-Specific Integrated Circuits (ASIC), in order to maintain flexibility for growth and design modifications.
The Navigator was designed to operate autonomously to enable the use of GPS for onboard navigation in high altitude space missions. With the exception of GPS signals, Navigator requires no external data (e.g., current time estimate, recent GPS almanac, or converged navigation filter estimate of the receiver dynamics).
By double buffering data up front in 1ms blocks, data can be processed as it is acquired. A discrete Fourier transform (DFT) is used to calculate the 1ms correlations, significantly reducing computing time. Computational efficiency is optimized and tradeoffs among sampling rate, data format, and data-path bit rate are carefully weighed in order to increase performance of the algorithm.
In addition, the Navigators hardware-independent receiver software includes both a hardware interface to perform low-level functions as well as basic navigation. Onboard orbit determination and accurate state estimation/propagation during periods with no GPS access are accomplished by integration with the GPS Enhanced Onboard Navigation System (GEONS).
Exploiting the properties of Fourier transform in a massively parallel search for the GSP signal, the Navigator has been tested and proven capable of acquiring signals at 25dB-Hz and below.

Lunar Surface Navigation System
NASAs reverse-ephemeris lunar navigation system is a concept for determining position on the lunar surface based on known orbits of satellites. In conventional GPS navigation systems, the GPS satellite transmits ephemeris data to a receiver on earth for determining position at the receiver location. Whereas for the reverse-ephemeris approach the receiver becomes the transmitter, and the satellite instead serves more as a fixed reference position with a known ephemeris. This simplifies the satellite requirements and also mitigates potential navigational disruptions that can otherwise arise in navigation systems that utilize satellite-based communications, for example from interference, jamming, etc.
The design consists of lunar surface S-Band (2,400 2,450 MHz) 10 W transceivers ranging with analog translating transponders on a three-satellite constellation in frozen elliptical orbits to provide continuous coverage with service to 300 simultaneous users over 1.8 MHz of bandwidth at the transponder. Digital bases systems are possible too. As compared to GPS-based navigation requiring four or more satellites costing 100s of millions of dollars, the new NASA concept is based on using only three smallsats.

Space Traffic Management (STM) Architecture
As ever larger numbers of spacecraft seek to make use of Earth's limited orbital volume in increasingly dense orbital regimes, greater coordination becomes necessary to ensure these spacecraft are able to operate safely while avoiding physical collisions, radio-frequency interference, and other hazards. While efforts to date have focused on improving Space Situational Awareness (SSA) and enabling operator to operator coordination, there is growing recognition that a broader system for Space Traffic Management (STM) is necessary. The STM architecture forms the framework for an STM ecosystem, which enables the addition of third parties that can identify and fill niches by providing new, useful services. By making the STM functions available as services, the architecture reduces the amount of expertise that must be available internally within a particular organization, thereby reducing the barriers to operating in space and providing participants with the information necessary to behave responsibly. Operational support for collision avoidance, separation, etc., is managed through a decentralized architecture, rather than via a single centralized government-administered system.
The STM system is based on the use of standardized Application Programming Interfaces (API) to allow easier interconnection and conceptual definition of roles to more easily allow suppliers with different capabilities to add value to the ecosystem. The architecture handles basic functions including registration, discovery, authentication of participants, and auditable tracking of data provenance and integrity. The technology is able to integrate data from multiple sources.

Affordable Vehicle Avionics (AVA)
Significant contributors to the cost of launching nano- and micro-satellites to orbit are the costs of software, and Guidance, Navigation and Control (GNC) avionics systems that steer, navigate and control the launch vehicles, sequence stage separation, deploy payloads, and pass data to Telemetry. The high costs of these GNC avionics systems are due in part to the current practice of developing unique, custom, single-use hardware and software for each launch, and requiring high-precision measurements of position and attitude states. NASA Ames Research Center has developed and tested a low-cost avionics system prototype called Affordable Vehicle Avionics (AVA). AVA integrates a low-cost moderately-precise sensor suite with an advanced error-correcting software package to provide GNC for space launch vehicles in a package smaller than a multilayer sandwich (100 mm x 120 mm x 69 mm; 4in x 4.7in x 2.7in), and with a mass of less than 0.84kg (2lbs). The invention provides a common suite of avionics components and demonstration software that deliver affordable, capable GNC with flexible I/O which is applicable to a variety of nano/micro-sat launch vehicles at less than 10 percent of the cost to procure current state-of-the-art GNC avionics. Affordable Vehicle Avionics' (AVA's) approach to drastically reduce costs includes: (1) use of low-cost "tactical-grade" Commercial-off-the-Shelf MicroElectroMechanical Systems (MEMS) inertial measurement unit, wherein adequate navigation precision is achieved by fusing outputs from a Global Positioning System receiver, inertial sensors and a magnetic field vector sensor in an extended Kalman filter formulation that corrects inertial sensor biases; (2) a streamlined "cookbook" approach to define an effective process for launch vehicle developers to design, simulate, verify and support assembly, integration and testing of their SLVs, driven by high-fidelity six degrees of freedom SLV simulations and real-time hardware-in-loop tests to validate guidance, navigation and control for early test flights.
Development Status:
As of spring 2020, AVA has flown twice in its current configuration on a suborbital platform. Its navigation and control functions were successfully demonstrated for roll-rate control within a tight deadband onboard the first flight test, and it successfully issued attitude pointing commands to a failed reaction control subsystem and it issues issued a rocket-motor ignition command on a second flight test. To date, failure of SLV components other than AVA (e.g., electrical power) has precluded demonstration of navigation and control of an orbital or sub-orbital launch system, which remains to be demonstrated.
AVA development was accomplished using a single magnetometer-based magnetic field vector sensor to provide attitude observability during free-fall (inter-stage coast periods). Therefore, the current tested AVA configuration is susceptible to magnetic/electric fields produced by other components and payloads onboard the SLV, so care must be exercised to either mount AVA well away from sources of such fields and or to incorporate magnetic/electric field barriers on field emitters if separation from emitters is inadequate. Also, licensees may wish to provide new AVA inputs from a pair of external horizon sensors to provide more accurate attitude navigation during coast phases of the SLV mission.

Spacecraft to Remove Orbital Debris
An approach to mitigating the creation of additional orbital debris is to remove the sources of future medium debris by actively removing large spent objects from congested orbits. NASA has introduced the ADRV, an efficient and effective solution to remove large debris from LEO such as spent rocket bodies and non-functional satellites. The concept yields a single use, low-cost, lightweight, high mass fraction vehicle that enables the specific removal of large orbital debris (1000 - 4000 kg mass, 200 - 2000 km altitude, and 20 98-degree inclination). The ADRV performs rendezvous, approach, and capture of non-cooperative tumbling debris objects, maneuvering of the mated vehicle, and controlled, targeted reposition or deorbit of the mated vehicle. Due to its small form factor, up to eight ADRVs can be launched in a single payload, enabling high impact orbital debris removal missions within the same inclination group.
Three key technologies were developed to enable the ADRV: - 1) The spacecraft control system (SCS) is a guidance, navigation, and control system that provides vehicle control during all phases of a mission; - (2) The debris object characterization system (DOCS) characterizes movement and capture of non-cooperative targets; and - (3) The capture and release system (CARS) allows the vehicle to capture and mate with orbital debris targets. These technologies can improve the current state-of-the-art capabilities of automated rendezvous and docking technology significantly for debris objects with tumbling rates up to 25 degrees per second. This approach leverages decades of spaceflight experience while automating key mission areas to reduce cost and improve the likelihood of success.