Room temperature oxygen sensors
sensors
Room temperature oxygen sensors (TOP2-300)
Small, low-cost, low-power, mass-producible oxygen sensors with room temperature operation
Overview
Oxygen (O2) sensors are used in a wide array of applications, including electronic fuel injection and emissions control in the automotive industry, for monitoring oxygen levels in controlled environments, such as in space craft or space suits, for oxygen monitoring in medical and pharmaceutical applications, and many more. Traditional oxygen sensors, including potentiometric and amperometric sensor designs, have significant drawbacks, as metal oxide gas sensors require high temperature operation of about 300C, and suffer from high power consumption. NASA Ames Research Center has developed novel oxygen sensors made of a hybrid material comprising graphene and titanium dioxide (TiO2) that is capable of detecting O2 gas at room temperature and ambient pressure. The sensors have fast response and recovery times and can also be used to detect ozone. The sensors can be integrated into wearable-sized Internet of Things (IoT) devices.
The Technology
NASA Ames has developed very small-sized oxygen sensors made of a graphene and titanium dioxide (TiO2) hybrid material. With ultraviolet (UV) illumination, these sensors are capable of detecting oxygen (O2) gas at room temperature and at ambient pressure. The sensors are able to detect oxygen at concentrations ranging from about 0.2% to about 10% by volume under 365nm UV light, and at concentrations ranging from 0.4% to 20% by volume under short wave 254nm UV light. These sensors have fast response and recovery times and can also be used to detect ozone.
This unique room temperature O2 sensor provides significant advantages in O2 sensing applications, especially those applications where high operating temperature requirements cannot be met, or would result in inefficient manufacturing processes. Since graphene is not intrinsically responsive to O2, and TiO2 is not responsive to oxygen at room temperature, the materials are first synthesized as a hybrid material. The synthesized graphene- TiO2 hybrid material is then ultrasonicated and then drop-casted onto a series of Interdigitated Electrodes (IDE) to form the sensors.
Ultrasonication ensures effective charge transfer at the graphene- TiO2 interphase. The graphene and the titanium dioxide may be present in the composite material in different ratios to ensure optimal oxygen detection. It is the combination of graphene with TiO2 that yields a semiconducting material capable of O2 sensing at room-temperature operation.
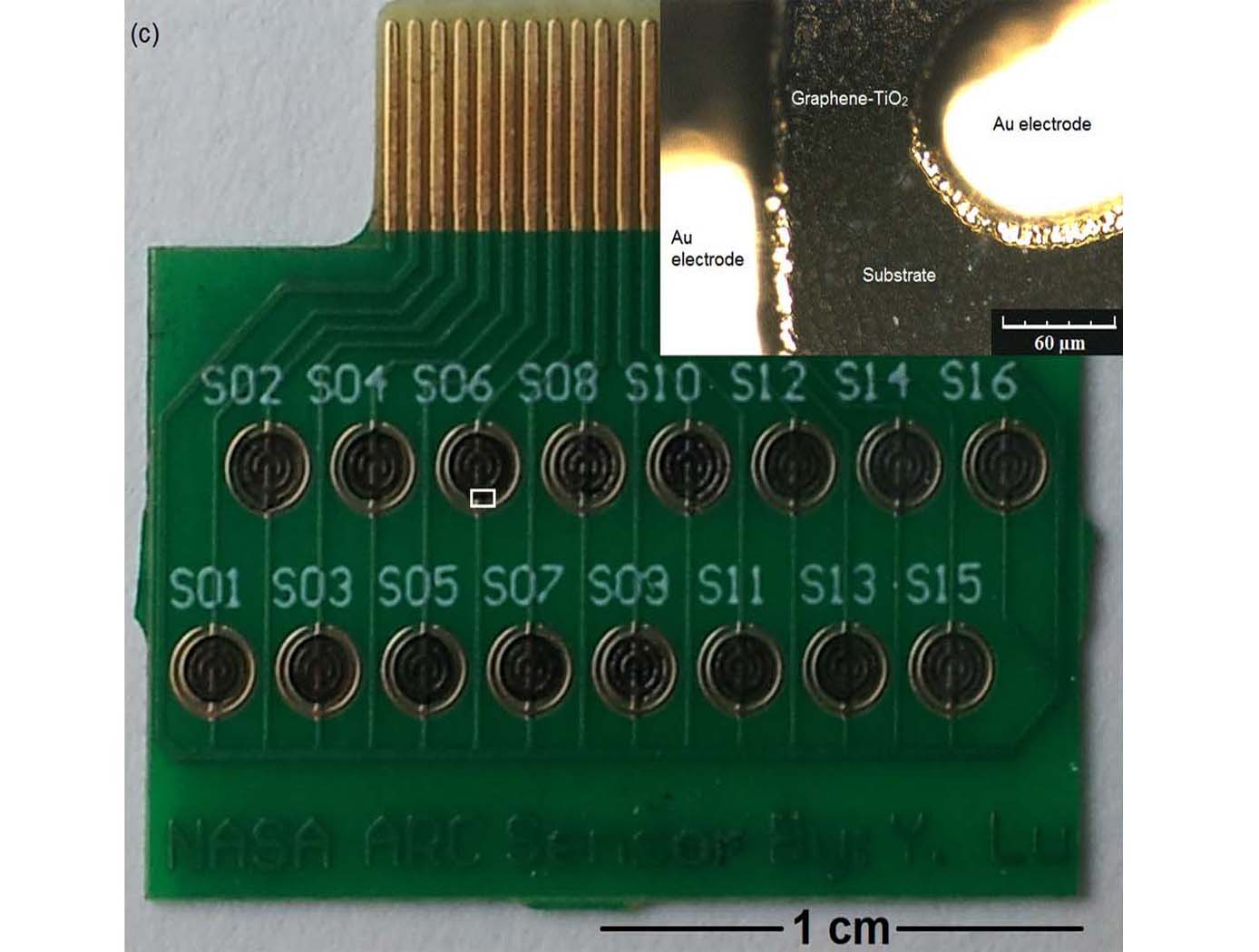
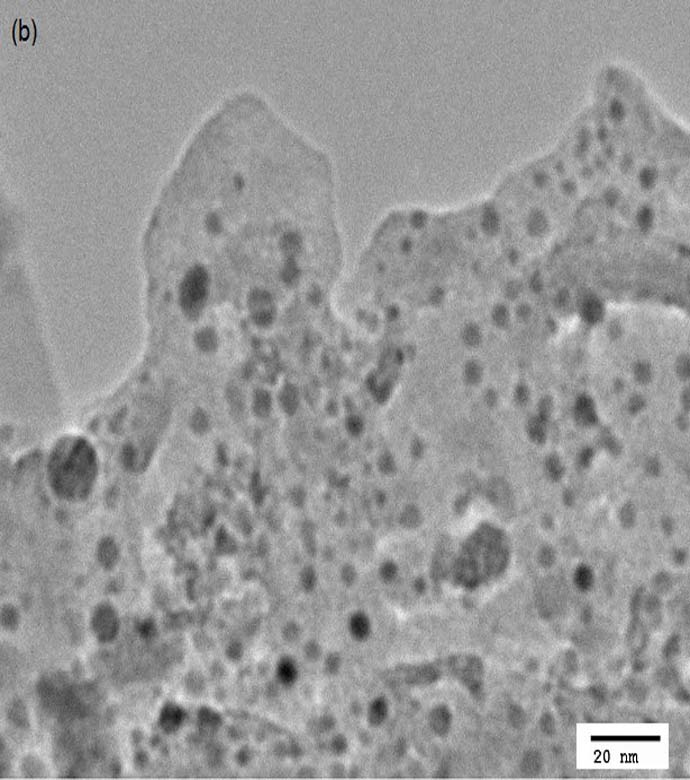
Benefits
- Composite hybrid graphene-TiO2 material allows O2 sensing at room-temperature operation, unlike traditional metal-oxide sensors
- Able to detect oxygen at concentrations ranging from about 0.2% to about 10% by volume under 365nm UV light, and at concentrations ranging from 0.4% to 20% by volume under short wave 254nm UV light
- Fast response and recovery times
- Small footprint that is one tenth (10%) the size current commercial O2 sensors, and has potential to be integrated in wearable-size IoT devices
- Mass producible: the sensor chip has the potential to scale to thousands or millions of units relatively easily and inexpensively via automated wafer-scale manufacturing processes
- Low-cost and ease of manufacturing: lower cost than current O2 sensors, as it is chip-based and can be manufactured via wafer scale fabrication in low-cost automated manufacturing processes to produce multiple chips on a single wafer
- May be integrated into an artificial intelligence device
Applications
- Oxygen sensor manufacturers
- Automotive combustion control and emissions control applications
- Chemical sensing
- Hybrid material development
- Spacecraft cabin
- Environmental monitoring
- Medical applications
- Food processing industry
- Steel and cement production industry
- Laboratory safety
- Electronic fuel injection industry
- Space suits and helmets
Tags:
|
Similar Results

Gas Sensors Based on Coated and Doped Carbon Nanotubes
A typical sensor device includes a set of interdigitated microelectrodes fabricated by photolithography on silicon wafer or an electrically insulating substrate. In preparation for fabricating the SWCNT portion of such a sensor, a batch of treated (coated or doped) SWCNTs is dispersed in a solvent. The resulting suspension of SWCNTs is drop-deposited or injected onto the area containing the interdigitated electrodes. As the solvent evaporates, the SWCNTs form a mesh that connects the electrodes. The density of the SWCNTs in the mesh can be changed by varying the concentration of SWCNTs in the suspension and/or the amount of suspension dropped on the electrode area. To enable acquisition of measurements for comparison and to gain orthogonality in the sensor array, undoped SWCNTs can be similarly formed on another, identical set of interdigitated electrodes. Coating materials tested so far include chlorosulfonated polyethylene. Dopants that have been tested include Pd, Pt, Au, Cu and Rh nanoparticle clusters. To date, the sensor has been tested for NO2, NH3, CH4, Cl2, HCl, toluene, benzene, acetone, formaldehyde and nitrotoulene.

Carbon Dioxide Gas Sensors
Current bulk or thick film solid electrolyte CO2 sensors are expensive, difficult to batch fabricate, and large in size. In contrast, this new amperometric, solid-state, oxide-based electrolyte CO2 microsensor is affordable, easy to fabricate, and is so small that it could easily be integrated onto a substrate the size of a postage stamp.
The basic composition of the sensor is identical to a previously designed NASA Glenn technology in which a solid electrolyte of Na3Zr2Si2PO12 is deposited between interdigitated electrodes on an alumina substrate and is covered by Na2CO3/BaCO3. Unlike its predecessor, however, this innovation includes an additional layer of nanocrystalline SnO2 sol gel, an electron donor type (N-type) semiconductor, on top of the Na2CO3/BaCO3 . This new layer provides a greater number of electrons for reduction reaction at the working electrode to detect CO2. As a result, overall performance is enhanced, and this new state-of-the-art sensor has the ability to operate at temperatures as low as 375°C. This low temperature capability significantly decreases the amount of power required to operate the sensor, opening the door to a multitude of new applications that were previously unattainable.

Polymer Electrolyte-Based Ambient Temperature Oxygen Microsensor
Conventional ambient-temperature oxygen sensors are limited in various ways: optically based sensors can be expensive and challenging to manufacture; electrochemical cells with liquid electrolytes can have limited lifetimes and become leak sources; and both types of sensors are difficult to miniaturize. These problems are addressed with Glenn's novel ambient temperature oxygen microsensor, which is based on a Nafiontm polymer electrolyte, microfabricated using thin-film technologies. In the past, one drawback of Nafiontm film has been that it can lose conductivity when the moisture content in the film is too low, potentially affecting sensor operation. Glenn researchers devised a method to use certain salts to hold water molecules in the Nafiontm film structure at room temperature. The presence of these salts provides extra sites in the film to promote proton (H+) mobility, thus improving film conductivity and overall sensor performance, particularly in arid and high-temperature environments.
The innovative use of metal/metal oxide as the reference electrode enables miniaturization by eliminating the reference gas and sealing the reference electrode. The combination of interdigitized electrodes with the unique metal/metal oxide reference electrode permits sensor operation in either potentiometric or amperometric mode, as appropriate. In potentiometric mode, which measures voltage differences between working and reference electrodes in different gases, the voltage differences can be monitored with a voltmeter; however, the sensor itself does not need a power source. In room-temperature testing, the sensor achieved repeatable responses to 21 percent oxygen in nitrogen (using nitrogen as a baseline gas), and also detected oxygen from 7 to 21 percent, making Glenn's breakthrough technology usable for personal health monitoring as well as fire detection, fuel-leak detection, and environmental monitoring.

Solid State Carbon Dioxide (CO2) Sensor
The technology is a solid state, Carbon Dioxide (CO2) sensor configured for sensitive detection of CO2 having a concentration within the range of about 100 Parts per Million (ppm) and 10,000 ppm in both dry conditions and high humidity conditions (e.g., > 80% relative humidity). The solid state CO2 sensor achieves detection of high concentrations of CO2 without saturation and in both dynamic flow mode and static diffusion mode conditions. The composite sensing material comprises Oxidized Multi-Walled Carbon Nanotubes (O-MWCNT) and a metal oxide, for example O-MWCNT and iron oxide (Fe2O3) nanoparticles. The composite sensing material has an inherent resistance and corresponding conductivity that is chemically modulated as the level of CO2 increases. The CO2 gas molecules absorbed into the carbon nanotube composites cause charge-transfer and changes in the conductive pathway such that the conductivity of the composite sensing material is changed. This change in conductivity provides a sensor response for the CO2 detection. The solid state CO2 sensor is well suited for automated manufacturing using robotics and software controlled operations. The solid state CO2 sensor does not utilize consumable components or materials and does not require calibration as often as conventional CO2 sensors. Since the technology can be easily integrated into existing programmable electronic systems or hardware systems, the calibration of the CO2 sensor can be automated.

Gas Composition Sensing Using Carbon Nanotube Arrays
An array of carbon nanotubes (CNTs) in a substrate is connected to a variable-pulse voltage source. The CNT tips are spaced appropriately from the second electrode maintained at a constant voltage. A sequence of voltage pulses is applied and a pulse discharge breakdown threshold voltage is estimated for one or more gas components, from an analysis of the current-voltage characteristics. Each estimated pulse discharge breakdown threshold voltage is compared with known threshold voltages for candidate gas components to estimate whether at least one candidate gas component is present in the gas. The procedure can be repeated at higher pulse voltages to estimate a pulse discharge breakdown threshold voltage for a second component present in the gas.
The CNTs in the gas sensor have a sharp (low radius of curvature) tip; they are preferably multiwall carbon nanotubes (MWCNTs) or carbon nanofibers (CNFs), to generate high-strength electrical fields adjacent to the current collecting plate, such as a gold plated silicon wafer or a stainless steel plate for breakdown of the gas components with lower voltage application and generation of high current. The sensor system can provide a high-sensitivity, low-power-consumption tool that is very specific for identification of one or more gas components. The sensors can be multiplexed to measure current from multiple CNT arrays for simultaneous detection of several gas components.