Search
Power Generation and Storage
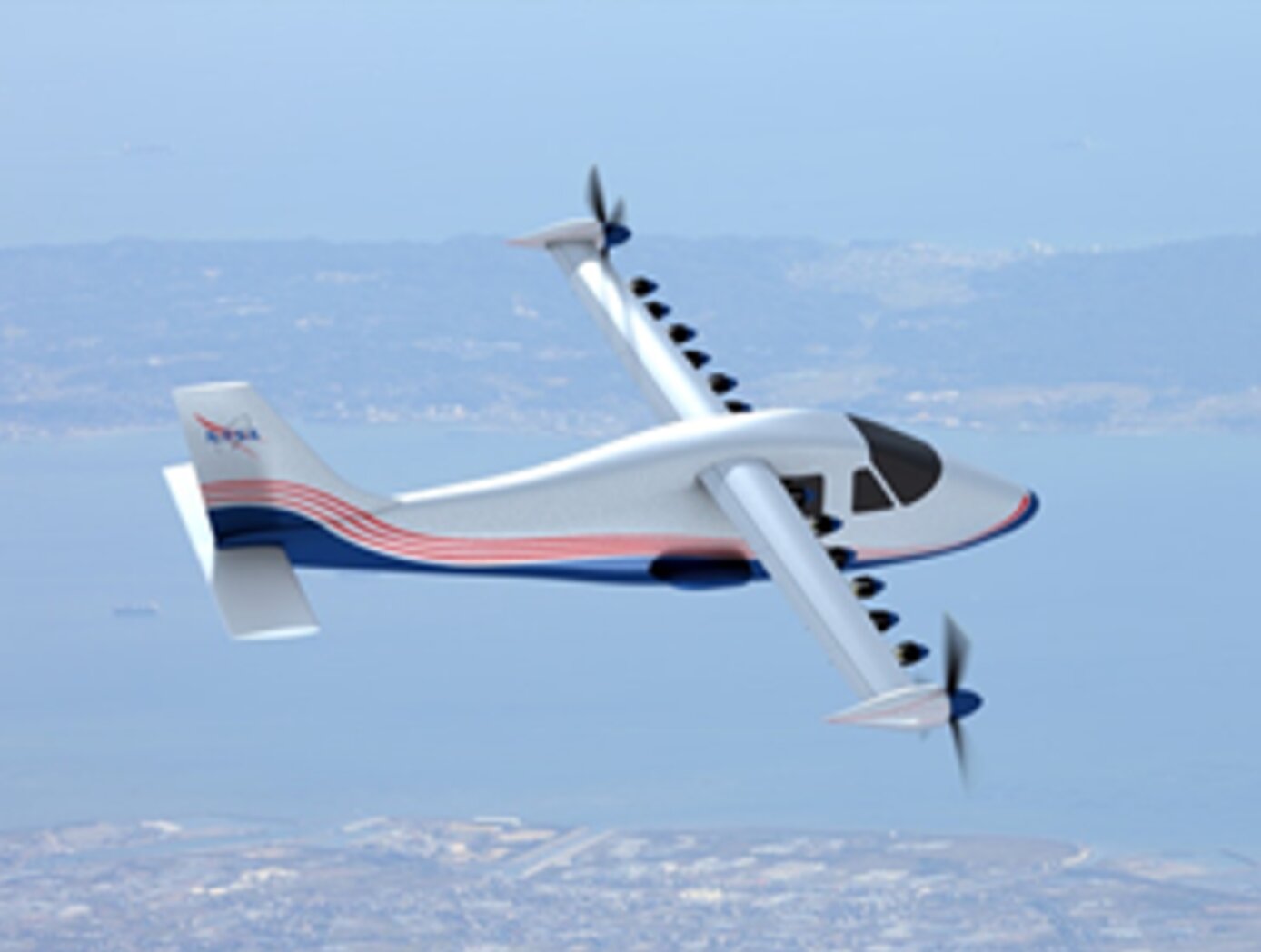
Solid-State Lithium-Sulfur Battery Tech Portfolio
The SABERS innovators developed novel lithium-sulfur designs, including sulfur-selenium on graphene cathodes, and lightweight bipolar plate stacking and packaging designs. SABERS is unique in several aspects: it deploys graphene-based manufacturing processes for the cathode and bipolar plates, and it uses a solid-state electrolyte in place of the liquid electrolyte found in other lithium-sulfur battery designs. The team has achieved energy densities over 500 W-hr/kg, and further improvements are expected. SABERS can meet the high-power requirements needed for aircraft take-off. SABERS is lightweight, safe, robust, and reliable. Furthermore, its manufacturing processes are scalable and environmentally friendly. Coin cell and pouch prototypes have been demonstrated to date. Development efforts continue and new portfolio innovations are expected. Major component technologies in SABERS include the following (as listed here and shown in the figure below). <ul>
<li>S/Se Cathode – Sulfur/Selenium on graphene scaffold
(LAR-19556-1, LEW-20228-1)
<li>Solid Electrolyte – Solid-state electrolyte composites
(LEW-20445-1)
<li>Bipolar Stack – Graphene plates
(LAR-20257-1)
<li>Li-Metal Anode
(Proprietary, under development)
<li>Packaging
(Proprietary, under development)</ul>
Robust computational models have been developed to support the battery materials design and are available to licensees to evaluate and optimize different materials combinations and performance targets.
sensors
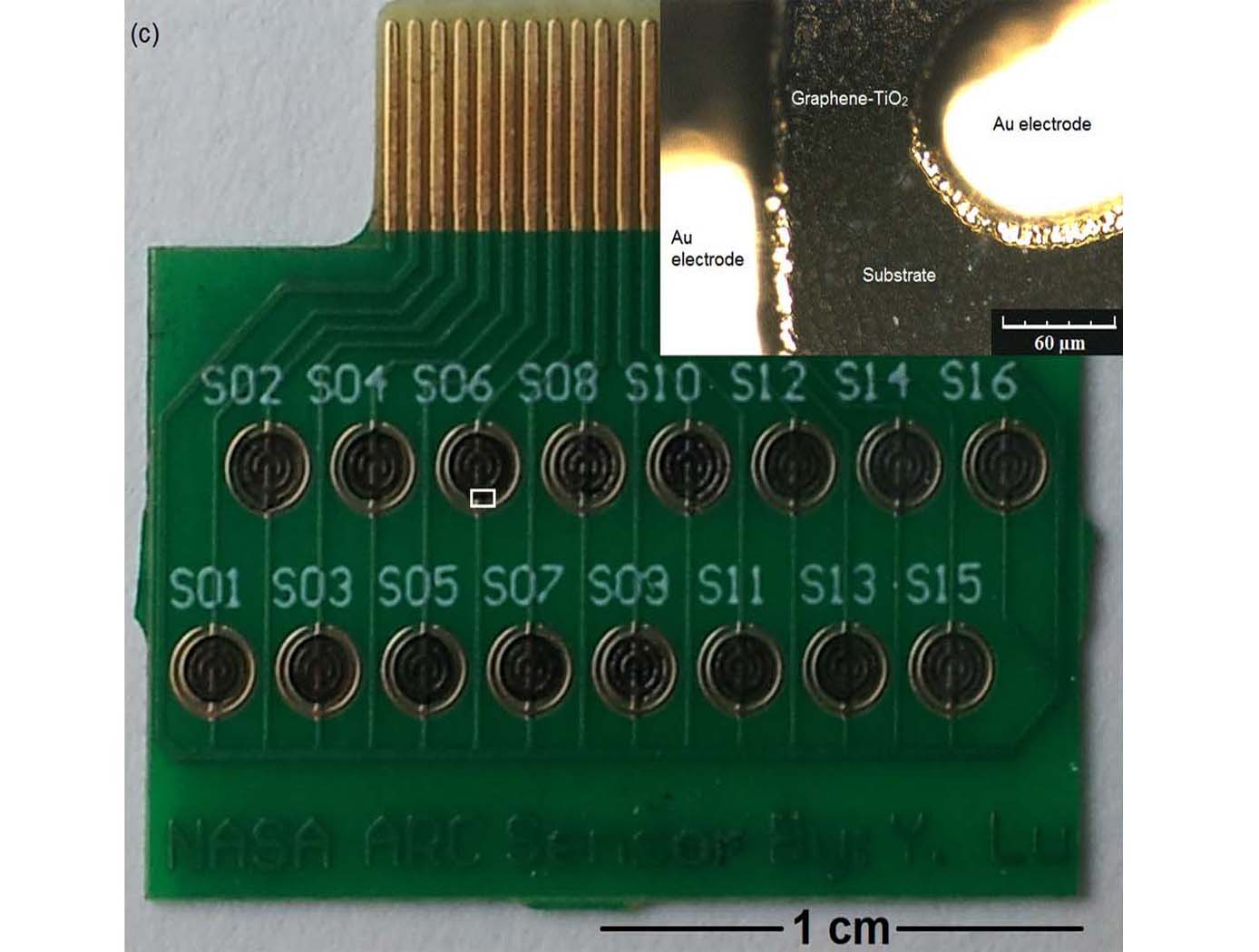
Room temperature oxygen sensors
NASA Ames has developed very small-sized oxygen sensors made of a graphene and titanium dioxide (TiO<sub>2</sub>) hybrid material. With ultraviolet (UV) illumination, these sensors are capable of detecting oxygen (O<sub>2</sub>) gas at room temperature and at ambient pressure. The sensors are able to detect oxygen at concentrations ranging from about 0.2% to about 10% by volume under 365nm UV light, and at concentrations ranging from 0.4% to 20% by volume under short wave 254nm UV light. These sensors have fast response and recovery times and can also be used to detect ozone.
This unique room temperature O<sub>2</sub> sensor provides significant advantages in O<sub>2</sub> sensing applications, especially those applications where high operating temperature requirements cannot be met, or would result in inefficient manufacturing processes. Since graphene is not intrinsically responsive to O<sub>2</sub>, and TiO<sub>2</sub> is not responsive to oxygen at room temperature, the materials are first synthesized as a hybrid material. The synthesized graphene- TiO<sub>2</sub> hybrid material is then ultrasonicated and then drop-casted onto a series of Interdigitated Electrodes (IDE) to form the sensors.
Ultrasonication ensures effective charge transfer at the graphene- TiO<sub>2</sub> interphase. The graphene and the titanium dioxide may be present in the composite material in different ratios to ensure optimal oxygen detection. It is the combination of graphene with TiO2 that yields a semiconducting material capable of O<sub>2</sub> sensing at room-temperature operation.
Materials and Coatings
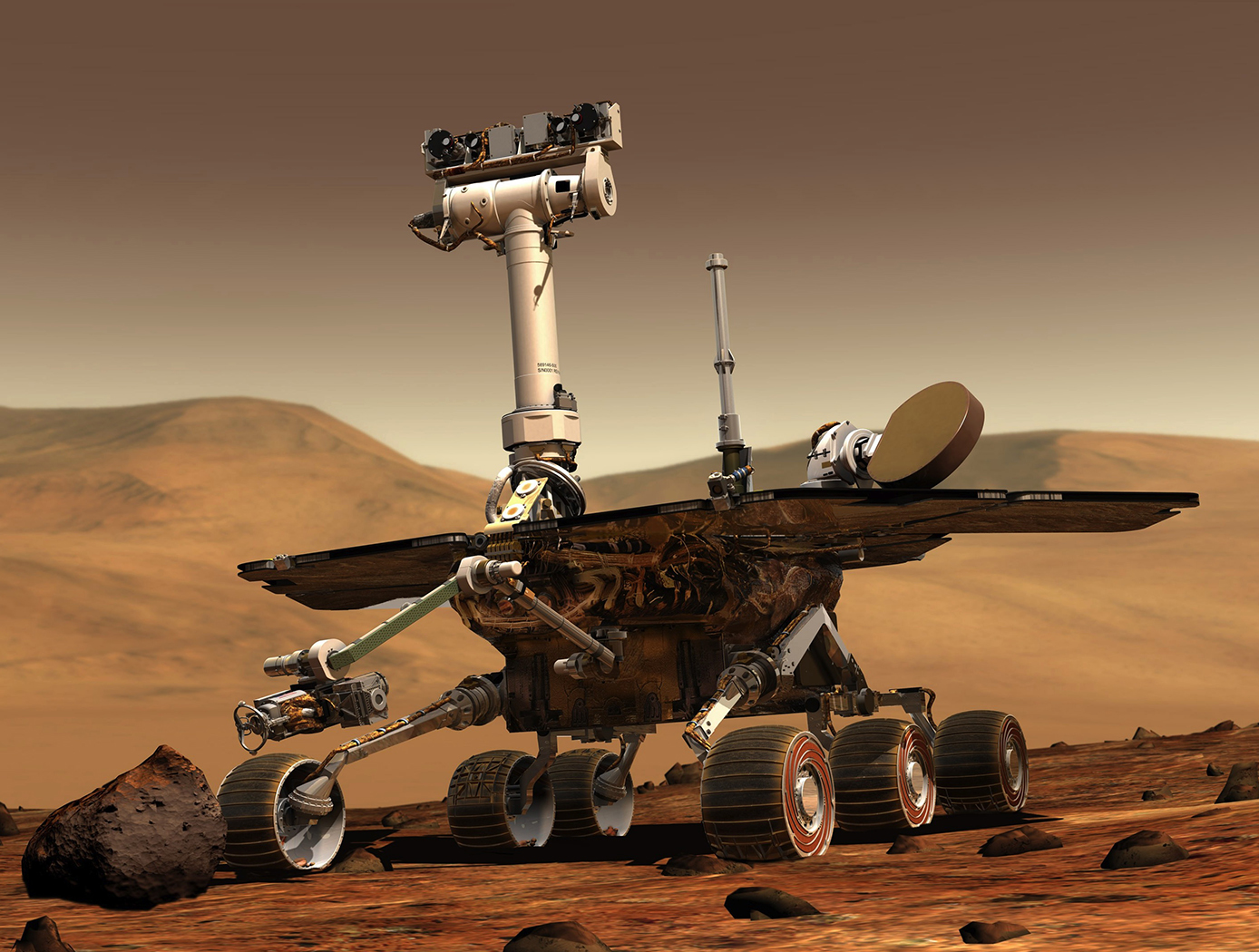
New Methods in Preparing and Purifying Nanomaterials
Sometimes called white graphite, affordable and plentiful hBN possesses the same kind of layered molecular structure as graphite. In graphite, this structure has allowed next-generation nanomaterials like carbon nanotubes and graphene to be produced. With hBN, however, the process of converting the substance into boron nitride nanotubes (BNNT) has been too difficult to yield commercial quantities. Glenn innovators have created several new methods that could enable greater adoption of this unique nanomaterial. In the initial stage, the starter reactant is mixed with a selected set of chemicals (a metal chloride, for example) and an activation agent (such as sodium fluoride). This mixture causes hBN to become less resistant to intercalation. The intercalated product can then be exfoliated by heating the material in air, and giving the material a final rinse with a liquid-phase ferric chloride salt to dissolve any embedded impurities without damaging its internal structure. These efficiently exfoliated nanomaterials can be used to form advanced composite materials (e.g., layered with aluminum oxide to form hBN/alumina ceramic composites). Nanomaterials fabricated from hBN can also take advantage of the material's unique combination of being an electrical insulator with high thermal conductivity for applications ranging from microelectronics to energy harvesting. Glenn's innovations have enabled a significantly improved matrix composite material with the potential to make a significant impact on the commercial materials market.