Damage Simulation Tool For Composite Laminates
instrumentation
Damage Simulation Tool For Composite Laminates (LAR-TOPS-317)
Faster and more detailed composite damage simulation results
Overview
This simulation tool is designed for progressive failure simulation in composite laminates. This simulation tool provides accurate results equivalent to those of a high fidelity model without the associated cost and time.
In doing this, the tool will generate more rapid results compared to high fidelity modeling, be a viable evaluation method for industry and allow for consideration of more complex problems that exceed the capability of currently available simulation methods within academia.
The simulation tool is in the form of a subroutine for an enriched finite element. A user would create a numerical model with the enriched element in an initially low fidelity form. The enriched elements then have the ability to increase fidelity locally to suit an ongoing damage process if necessary.
The Technology
The simulation combines existing fracture mechanics based damage propagation techniques with a discrete approach to modeling discontinuities in finite elements. Additionally, the use of an advanced laminate theory recovers deformation and stress information that would normally require a high fidelity model.
To accomplish this, the same theoretic and analytical concepts that a high fidelity numerical simulation tool utilizes for laminate damage simulation are placed in the context of a low fidelity finite element. In taking this approach, a laminate can be modeled as a single layer low fidelity shell mesh that has the ability to locally increase fidelity and represent a delamination based damage process but only if it is determined that one should occur.
The numerical simulation tool's performance has been validated against numerical benchmarks as well as experimental data.

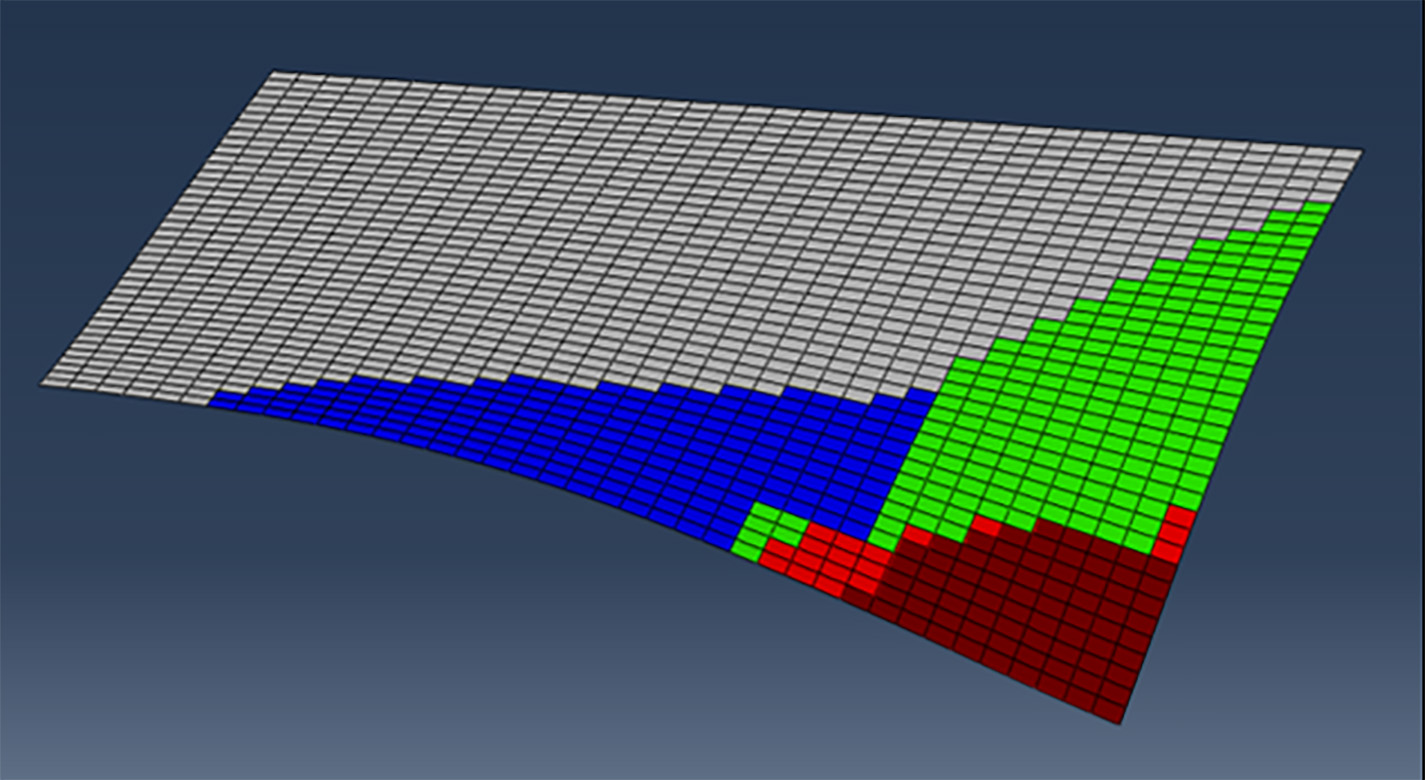
Benefits
- Viable for manufacturing and industrial users - not just academia
- Enables faster simulation of low impact fracture damage in composite laminates
- Expands understanding of low impact fracture damage in composite laminates
- Enables simulation of more complex problems than currently possible
Applications
- Academic research and development of more damage tolerant composite laminates
- Industrial design and damage tolerance validation for manufacturing of composite laminate components
Tags:
|
Similar Results

Fabrication of Fiber-Metal Laminates with Non-Autoclave Processes
The FMLs resulting from the NASA process have similar properties to traditionally produced metal/composite hybrid laminates including, as compared to either the composite or metal only structures, improved load carrying capability, lighter weight, improved stiffness, improved impact resistance and damage tolerance, and improved permeation resistance. The NASA process can be applied to various FML types, including GLARE (glass, aluminum, epoxy), and TIGR (titanium, graphite). Typical manufacturing processes are costly and complex shapes are hard to produce, whereby the NASA process enables use of these kinds of laminates without an autoclave or press, thus increasing the size that can be produced and decreasing the cost.
The resin pathways in the foils enable connection between the plies that can improve the interlaminar strength of the final part. Functionally the NASA process creates resin columns in the transverse direction of the plies. NASA is working to optimize the final properties by varying the size and distribution of the pathways.

Advanced Isothermally Produced Next-Gen Composites
Next generation aircraft are anticipated to be largely made with composite components, requiring significant increases in manufacturing rates of composites to meet the demand for a new fleet of aircraft. The higher rate manufacturing will require multiple advances, including rapid curing and lower processing temperatures. These requirements can be enabled by new processing methods such as isothermal rapidly cured composite parts.
NASA has developed materials and methods that meet those stringent requirements for high-rate manufacturing. The innovators have demonstrated at least two families of new resin formulations that meet the expected high-rate manufacturing needs. These new formulations have been engineered to be infused and cured at the same (i.e., isothermal) temperature, below that of commercially available materials. The materials can then be removed from the mold while still hot without distorting the shape, thereby reducing the processing times by eliminating the need for cooling to occur in the mold. After a post-cure process - which takes 4 hours or less and can be performed in batches - the mechanical properties of NASA's next-gen composites.
The related patent is now available to license. Please note that NASA does not manufacturer products itself for commercial sale.

Plasma Deposition of Metal in Composite Panels
NASA's plasma-deposition process provides the ability to tailor various properties while designing functional parts by selecting specific materials and processing parameters to meet the end goal. Specifically, the plasma process deposits metal particles that are heated as they travel axially at low velocity through an inert gas plasma. The accelerated powder particles become molten, strike the substrate fabric (uniaxial, biaxial, and multiaxial) and rapidly solidify, imparting very little heat to the substrate while forming a metal-to-fiber bond, as well as a metal-to-metal bond. The resulting metal-coated fabric is porous, so the polymer matrix can pass through the product precursor during the infusion process. The amount of metal deposited can be
controlled, as can the number of plies of fabric that are ultimately stacked to produce the preform for the polymer matrix infusion process. A variety of infusion processes can be utilized to prepare the FML, including resin transfer molding (RTM), resin film infusion (RFI), and vacuum-assisted resin transfer molding (VARTM). The tailorable aspect of the process allows for specific product design. By varying the combination of metal particle, fiber, fabric type, metal layer thickness, fabric direction, number of layers, polymer matrix resin, infusion process, and cure conditions, the characteristics of the final part can meet the needs of various applications.

Method of Non-Destructive Evaluation of Composites
Guided wavefield techniques require excitation of guided waves in a specimen via contact or noncontact methods (such as attached piezoelectric transducers or laser generation). The resulting wavefield is recorded via noncontact methods such as laser Doppler vibrometry or air-coupled ultrasound. If the specimen contains damage, the waves will interact with that damage, resulting in an altered wavefield (compared to the pristine case). When guided wave modes enter into a delaminated region of a composite the energy is split above/below delaminations and travels through the material between delaminations. Some of the energy propagates beyond the delamination and re-emerges as the original guided wave modes. However, a portion
of the wave energy is trapped as standing waves between delaminations. The trapped waves slowly leak from the delaminated region, but energy remains trapped for some time after the incident waves have propagated beyond the damage region.
Simulation results show changes in the trapped energy at the composite surface when
additional delaminations exist through the composite thickness. The results are a
preliminary proof-of-concept for utilizing trapped energy measurements to identify
the presence of hidden delaminations when only single-sided access is available to a
component/vehicle. Currently, no other single-sided field-applicable NDT techniques
exist for identifying hidden delamination damage.

AERoBOND: Large-scale Composite Manufacturing
This technology (AERoBOND) enables the assembly of large-scale, complex composite structures while maintaining predictable mechanical and material properties. It does so by using a novel barrier-ply technology consisting of an epoxy resin/prepreg material with optimal efficiency, reliability, and performance. The barrier-ply materials prevent excessive mixing between conventional composite precursors and stoichiometrically-offset epoxy precursors during the cure process by forming a gel early in the cure cycle before extensive mixing can occur. The barrier ply is placed between the conventional laminate preform and the stoichiometrically-offset ply or plies placed on the preform surface, thus preventing excessive mass transfer between the three layers during the cure process. In practice, the barrier ply could be combined with the offset ply to be applied as a single, multifunctional surfacing layer enabling unitized assembly of large and complex structures. The AERoBOND method is up to 40% faster than state-of-the-art composite manufacturing methods, allows for large-scale processing of complex structures, eliminates the potential for weak bond failure modes, and produces composites with comparable mechanical properties as compared with those prepared by co-cure.