AERoBOND: Large-scale Composite Manufacturing
manufacturing
AERoBOND: Large-scale Composite Manufacturing (LAR-TOPS-357)
A method for the reliable manufacturing of complex composite structures
Overview
NASA Langley Research Center has an improved method for making composite structures. Industrial composite manufacturing is primarily accomplished through three methods: co-cure, co-bond, and secondary bond processes. Co-cure produces predictable structures and joint properties but is costly and challenging to implement on large, complex structures. Co-bond and secondary bond processes have greater applicability to complex structures but produce unpredictable material interfaces and properties. NASAs AERoBOND technology presents a method for manufacturing composites at scale with the reliability of co-cure in a bonded assembly process. AERoBOND utilizes novel epoxy and barrier ply layers with optimized chemical and physical properties to enable the bonding of large, complex composites with the advantages of co-cure assembly but without the need for redundant fasteners, thus reducing assembly time and cost.
The Technology
This technology (AERoBOND) enables the assembly of large-scale, complex composite structures while maintaining predictable mechanical and material properties. It does so by using a novel barrier-ply technology consisting of an epoxy resin/prepreg material with optimal efficiency, reliability, and performance. The barrier-ply materials prevent excessive mixing between conventional composite precursors and stoichiometrically-offset epoxy precursors during the cure process by forming a gel early in the cure cycle before extensive mixing can occur. The barrier ply is placed between the conventional laminate preform and the stoichiometrically-offset ply or plies placed on the preform surface, thus preventing excessive mass transfer between the three layers during the cure process. In practice, the barrier ply could be combined with the offset ply to be applied as a single, multifunctional surfacing layer enabling unitized assembly of large and complex structures. The AERoBOND method is up to 40% faster than state-of-the-art composite manufacturing methods, allows for large-scale processing of complex structures, eliminates the potential for weak bond failure modes, and produces composites with comparable mechanical properties as compared with those prepared by co-cure.
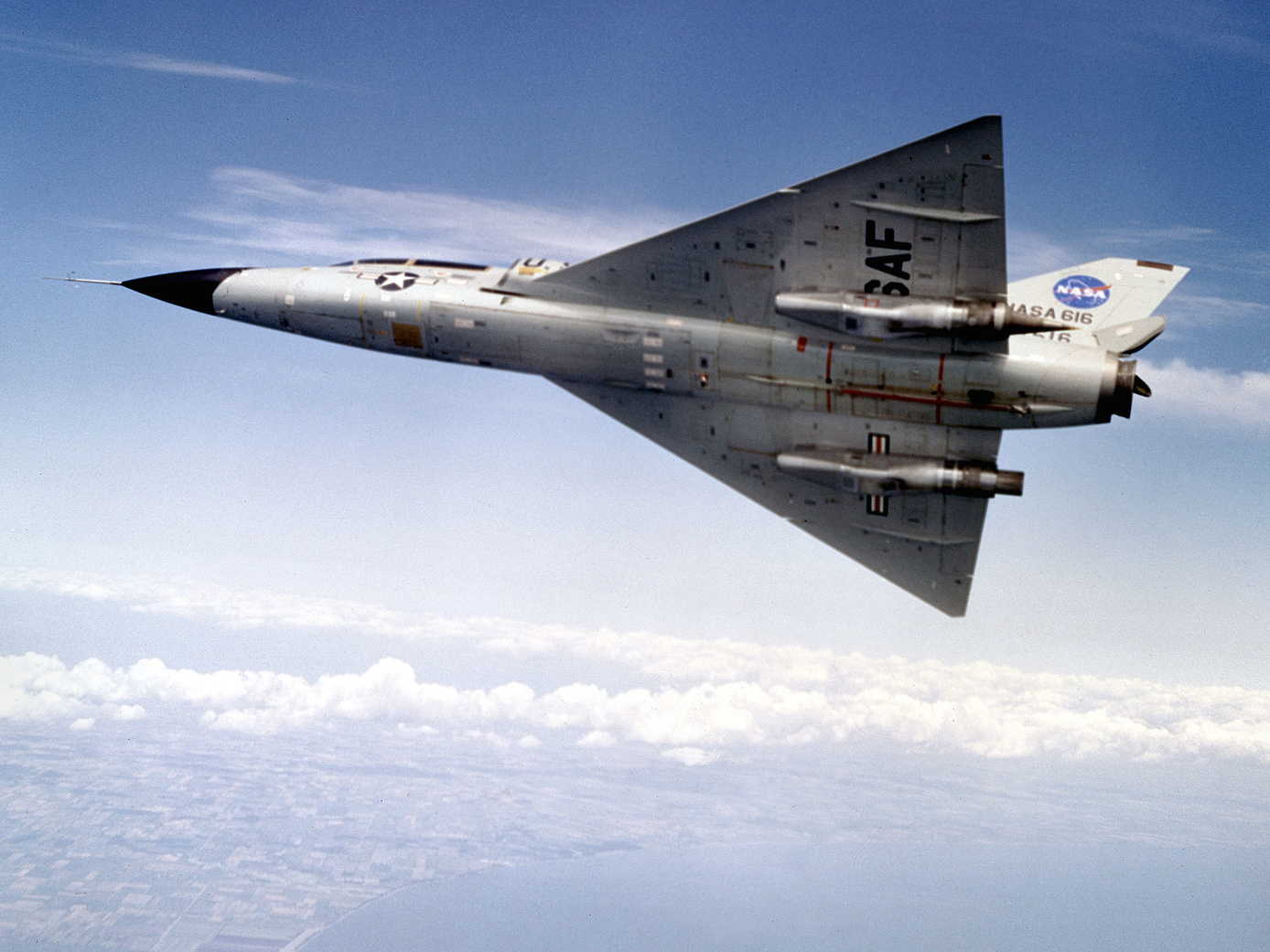
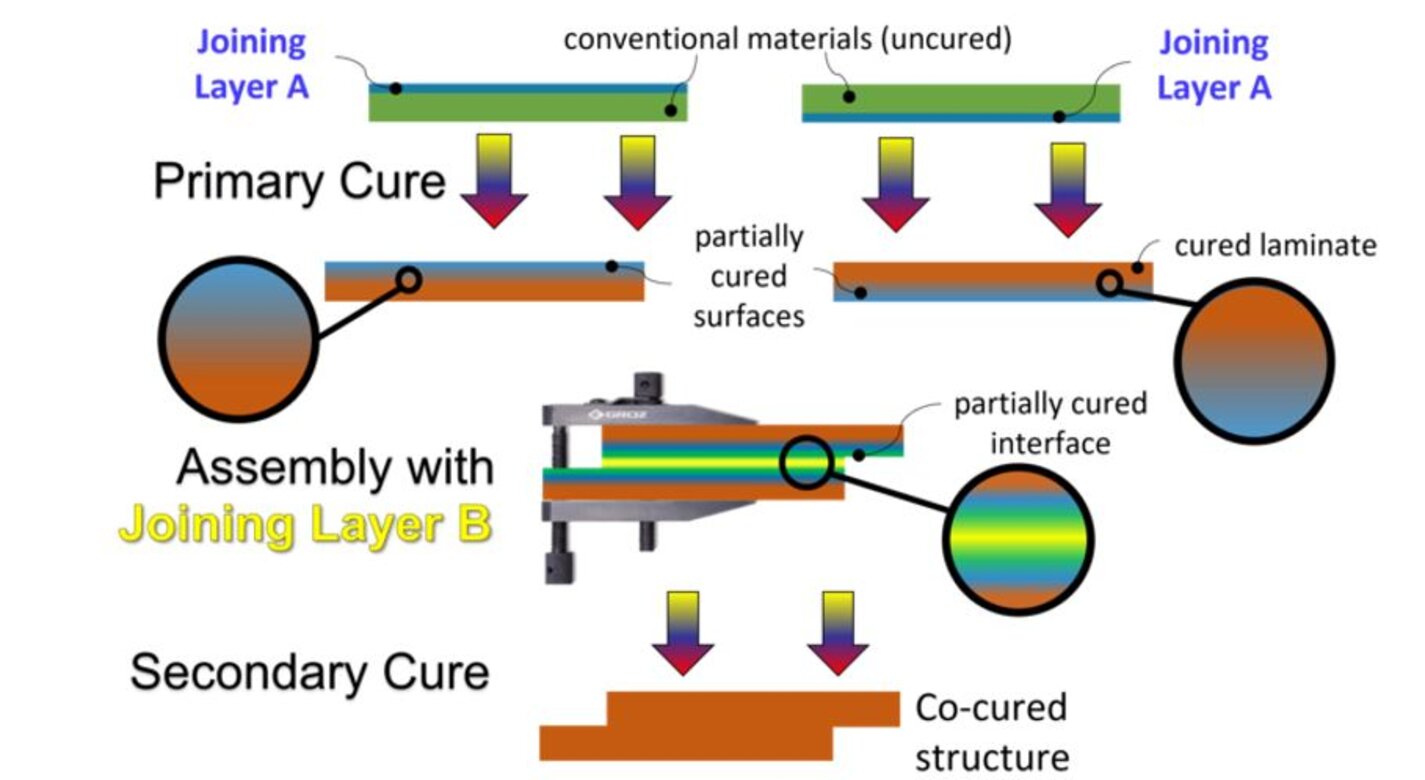
Benefits
- High Throughput: up to 40% faster than state-of-the-art composite manufacturing method
- Scalability: precursor materials are commercially available, and the process is readily scalable
- Reliability: eliminates the potential for weak bond failure modes that can occur in bonded assemblies
- Excellent Mechanical Properties: composites produced via AERoBOND have mechanical performance that is comparable with co-cure or co-bond equivalents
Applications
- Aerospace: composite airframe and fuselage assembly for ultra-efficient aircraft structures
- Marine: lightweight, damage-resistant hulls
- Wind Energy: wind turbine blades
- Automotive: fuel-efficient framing and parts
- Architecture: structural building components
Technology Details
manufacturing
LAR-TOPS-357
LAR-19860-1
LAR-18677-1
LAR-18677-1-CON
(1) Palmieri, F.L., Hudson, et.al., Latent cure epoxy resins for reliable joints in secondary-bonded composite structures. Composites Part B: Engineering, 231, 109603 (2022). (2)
Palmieri, F. L., Hudson, et. al., NASA Facts: AERoBOND Project. TACP Showcase. (2021). (3)
Palmieri, F. L., & Hudson, T. B. AERoBOND Project Summary. (2020). (4)
Palmieri, F.L., Hudson, et. al., Reduced Dependence on Redundant Fasteners in Secondary-Bonded Composite Structures Using Modified Epoxy Matrix Resins. 43rd Ann. Mtg of The Adhesion Society. No. NF1676L-34246. Feb.2020).
Similar Results

AERoBOND+ for Manufacturing Composite Structures
The AERoBOND and AERoBOND+ technologies are composite resin materials design innovations that enable new methods for composites joining and manufacturing. The resins are formulated with carefully selected off-set stoichiometries to delay/control the cure such that initial curing of individual components can be followed separately by joining/curing of components together. The ability to delay and control the co-cure joining step provides ease of manufacturing of multi-part composite structures, without compromising joint integrity. There are significant cost savings associated with eliminating fasteners and joint surface preparation steps. To date, the focus of the NASA development effort has been on novel epoxy-based prepreg formulations though other types of thermosets could be considered as well.
The AERoBOND+ innovation provides an added adhesive layer to the AERoBOND joint design to improve the ability to join composite surfaces when these surfaces are less tightly matched. Conventional adhesives, e.g., film, paste, etc., are employed. By including an adhesive between the offset stoichiometric prepreg plies, the adhesive can fill the gaps between the bonding surfaces while maintaining reflowable AERoBOND layer interfaces. Since all interfaces are reflowable, they are much more tolerant of surface contamination, thereby mitigating a primary challenge for conventional adhesive bonding.

3D Printed Polymer Aerogels
NASA GRC has developed several different 3D printing techniques to fabricate polymer aerogel structures; different techniques are suitable for different polymer aerogel formulations. In general, the methods entail forming a first liquid comprising a polymer precursor and cross-linker, forming a second liquid comprising a catalyst for reacting a polymer precursor to form a polymer, then 3D printing the aerogel structure with a mixture of the two liquids onto or into a medium.
Specifically, NASA's polymer aerogel 3D printing techniques include: direct syringe printing, dual-syringe printing with static mixing head, direct syringe printing of photocurable formulations, and a unique rapid prototyping method involving a sacrificial bath. The direct syringe process relies upon gelation to occur once the gel is extruded from the print head to the substrate. In the dual-syringe printing process, one syringe contains polyamic acid and the other contains a catalyst. These are mixed in a static mixing head to allow for curing/gelation upon extrusion. For direct syringe printing of photocurable polymer aerogel formulations, a UV pen and array follow the printed gel during extrusion to induce gelation. Finally, the rapid prototyping method uses a bath of highly viscosity, low shear gel that provides a low drag, flexible yet supportive medium in which to print the polymer aerogel. In other embodiments, a bath of polyamic acid is used while cross-linkers and catalysts are introduced via printing, or vice versa (polyamic acid is introduced via printing into a bath of cross-linkers and catalysts).
In addition to complex geometries, these layered 3D printing processes allow for the fabrication of chemically-bonded (cross-linked) structures composed of layers of different polymer aerogel formulations (i.e., fabrication of structures with tailored chemistries / properties). Previously, joining different polymer aerogel formulations into a single structure required the fabrication of different formulations using individual molds and joining them using an adhesive, potentially introducing weak fracture points.

Blocking/Deblocking Resin Systems
This technology enables the fabrication of co-cured structures without the need for an autoclave or oven large enough to contain the full-scale structure. Instead, sub-components can be prepared in a less expensive, smaller autoclave or oven with co-cure plys applied to the faying surfaces. A continuous, assembled structure can be prepared using a subsequent curing process in a heated press. The co-cured structures can be designed to meet FAA certification criteria for composite structures because no adhesive bond or mechanical fasteners are needed. The structure can be treated as a single, joint-free component.

Recyclable Feedstocks for Additive Manufacturing
NASA's new technique for generating recyclable feedstocks for on-demand additive manufacturing employs the high-yield reversibility of the Diels-Alder reaction between maleimide and furan functionalities, utilizing the exceedingly favorable interaction between specific chemical functionalities, often termed "click reactions" due to their rapid rate and high efficiency. Integration of these moieties within a polymer coating on epoxy microparticle enables reversible assembly into macroscopic, free-standing articles. This click chemistry can be activated and reversed through the application of heat. Monomer species can be used to incorporate these functionalities into polyimide materials, which provide excellent mechanical, thermal, and electrical properties for space applications. Copoly (carbonate urethane) has been shown to be a viable coating material in the generation of polymer-coated epoxy microparticle systems and is amenable to being processed through a variety of approaches (e.g., filaments and slurries for 3D printing, compression molding, etc.). The polymeric materials are grown from the surfaces of in-house fabricated epoxy microparticles. The thermal and mechanical properties of the microparticles can be readily tuned by changes in composition.
There are a number of potential applications for this NASA technology ranging from use of these materials for recyclable/repurpose-able articles (structural, decorative, etc.) to simple children's toys. More demanding uses such as for replacement parts in complex industrial systems are also possible. For long term space missions, it is envisioned that these feedstocks would be integrated into secondary spacecraft structures such that no additional concerns would be introduced due to in-space chemical reactions and no additional mass would be required.

Aerofoam
The Aerofoam composites have superior thermal and acoustic insulation properties when compared to conventional polyimide foams. In addition, they provide greater structural integrity than the fragile aerogel materials can provide independently. In general, polymer foams can provide excellent thermal insulation, and polyimide foams have the additional advantage of excellent high-temperature behavior and flame resistance compared to other polymer systems (they do not burn or release noxious chemicals). Incorporating aerogel material into the polyimide foam as described by this technology creates a composite that has been demonstrated to provide additional performance gains, including 25% lower thermal conductivity with no compromise of the structural integrity and high-temperature behavior of the base polyimide foam. The structural properties of Aerofoam are variable based on its
formulation, and it can be used in numerous rigid and flexible foams of varying densities.
Aerofoam has a number of potential commercial applications, including construction, consumer appliances, transportation, electronics, healthcare, and industrial equipment. In addition, these high-performance materials may prove useful in applications that require insulation that can withstand harsh environments, including process piping, tanks for transporting and storing hot or cold fluids, ship and boat building, and aerospace applications.