AERoBOND+ for Manufacturing Composite Structures
Materials and Coatings
AERoBOND+ for Manufacturing Composite Structures (LAR-TOPS-380)
Prepreg/adhesive layer design improves ability to join mating surfaces
Overview
The original AERoBOND technology was developed to enable co-cure joining and manufacturing of large composite structures without the use of fasteners. The AERoBOND technology provides novel prepreg and resin formulation designs to improve the joining and manufacturing of high-performance, large composite structures such as those used in aviation and aerospace applications. AERoBOND can also be used for many other prepreg-based composite structures, small and large, in many industrial applications. The joint quality can be exceptional, and the cost and efficiency of manufacturing is improved.
The new AERoBOND+ innovation is a next-generation innovation that widens the scope of potential applications of AERoBOND to include those where the mating surfaces are less than ideally matched. This new innovation is accomplished with critically designed resin/adhesive/prepreg formulations and layer designs across the joint between each of the faying surfaces.
The Technology
The AERoBOND and AERoBOND+ technologies are composite resin materials design innovations that enable new methods for composites joining and manufacturing. The resins are formulated with carefully selected off-set stoichiometries to delay/control the cure such that initial curing of individual components can be followed separately by joining/curing of components together. The ability to delay and control the co-cure joining step provides ease of manufacturing of multi-part composite structures, without compromising joint integrity. There are significant cost savings associated with eliminating fasteners and joint surface preparation steps. To date, the focus of the NASA development effort has been on novel epoxy-based prepreg formulations though other types of thermosets could be considered as well.
The AERoBOND+ innovation provides an added adhesive layer to the AERoBOND joint design to improve the ability to join composite surfaces when these surfaces are less tightly matched. Conventional adhesives, e.g., film, paste, etc., are employed. By including an adhesive between the offset stoichiometric prepreg plies, the adhesive can fill the gaps between the bonding surfaces while maintaining reflowable AERoBOND layer interfaces. Since all interfaces are reflowable, they are much more tolerant of surface contamination, thereby mitigating a primary challenge for conventional adhesive bonding.
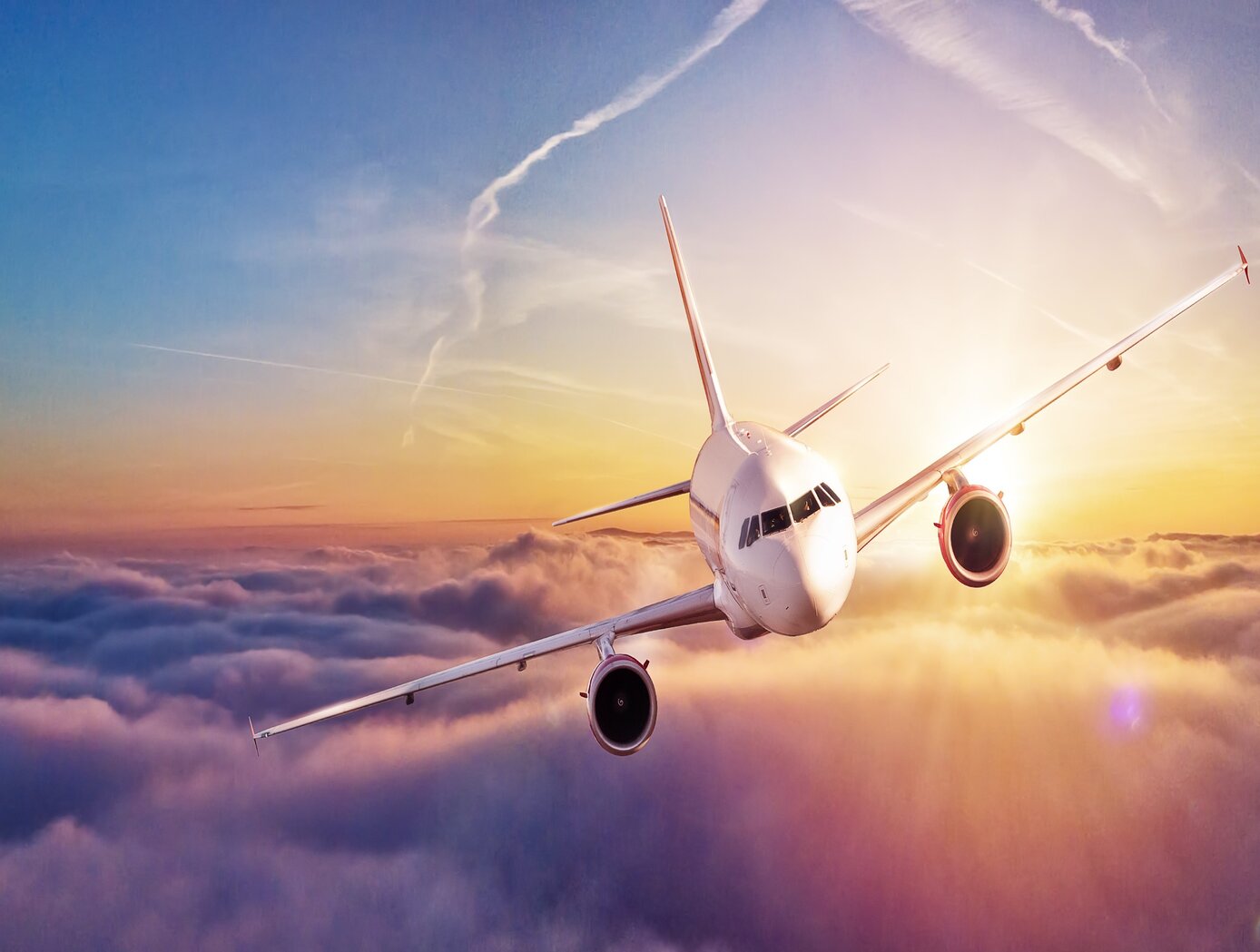
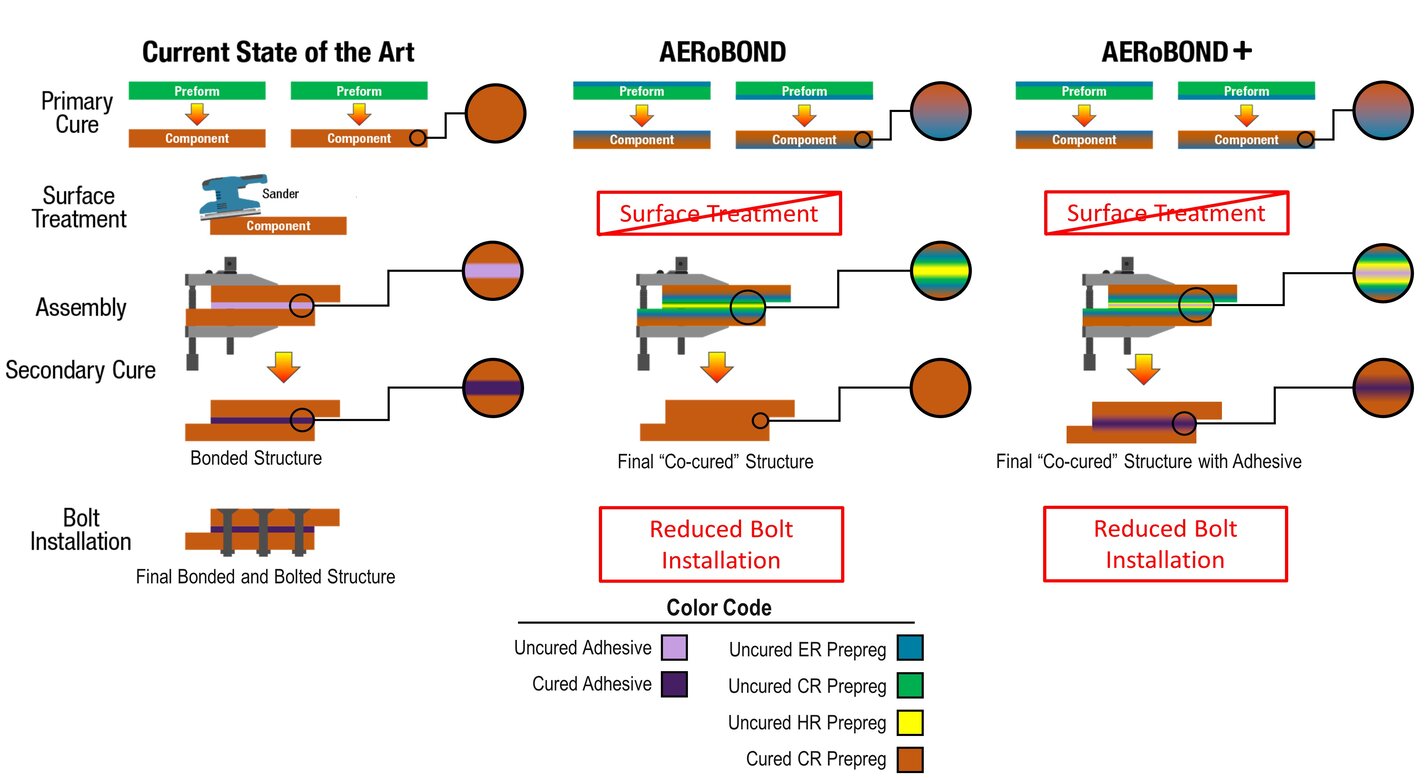
Benefits
- High mechanical integrity of composite joints, even for those with low tolerance for matching of faying surfaces
- Cheaper, faster composites joining and fabrication using low-precision tooling
- Resistance to surface contamination of the bonding surfaces
- A drop-in solution for composites joining
Applications
- AERoBOND+ has broad potential application for the manufacturing of composites used in all industries, including:
- Aerospace, including large assembled composite structures for airframes
- Marine, recreational and commercial construction
- Automotive
- Building and Construction
Technology Details
Materials and Coatings
LAR-TOPS-380
LAR-20315-1
LAR-19860-1
LAR-18677-1
LAR-18677-1-CON
Tags:
|
|
Related Links:
|
Similar Results

AERoBOND: Large-scale Composite Manufacturing
This technology (AERoBOND) enables the assembly of large-scale, complex composite structures while maintaining predictable mechanical and material properties. It does so by using a novel barrier-ply technology consisting of an epoxy resin/prepreg material with optimal efficiency, reliability, and performance. The barrier-ply materials prevent excessive mixing between conventional composite precursors and stoichiometrically-offset epoxy precursors during the cure process by forming a gel early in the cure cycle before extensive mixing can occur. The barrier ply is placed between the conventional laminate preform and the stoichiometrically-offset ply or plies placed on the preform surface, thus preventing excessive mass transfer between the three layers during the cure process. In practice, the barrier ply could be combined with the offset ply to be applied as a single, multifunctional surfacing layer enabling unitized assembly of large and complex structures. The AERoBOND method is up to 40% faster than state-of-the-art composite manufacturing methods, allows for large-scale processing of complex structures, eliminates the potential for weak bond failure modes, and produces composites with comparable mechanical properties as compared with those prepared by co-cure.

Advanced Isothermally Produced Next-Gen Composites
Next generation aircraft are anticipated to be largely made with composite components, requiring significant increases in manufacturing rates of composites to meet the demand for a new fleet of aircraft. The higher rate manufacturing will require multiple advances, including rapid curing and lower processing temperatures. These requirements can be enabled by new processing methods such as isothermal rapidly cured composite parts.
NASA has developed materials and methods that meet those stringent requirements for high-rate manufacturing. The innovators have demonstrated at least two families of new resin formulations that meet the expected high-rate manufacturing needs. These new formulations have been engineered to be infused and cured at the same (i.e., isothermal) temperature, below that of commercially available materials. The materials can then be removed from the mold while still hot without distorting the shape, thereby reducing the processing times by eliminating the need for cooling to occur in the mold. After a post-cure process - which takes 4 hours or less and can be performed in batches - the mechanical properties of NASA's next-gen composites.
The related patent is now available to license. Please note that NASA does not manufacturer products itself for commercial sale.

3D-Printed Composites for High Temperature Uses
NASA's technology is the first successful 3D-printing of high temperature carbon fiber filled thermoset polyimide composites. Selective Laser Sintering (SLS) of carbon-filled RTM370 is followed by post-curing to achieve higher temperature capability, resulting in a composite part with a glass transition temperature of 370 °C.
SLS typically uses thermoplastic polymeric powders and the resultant parts have a useful temperature range of 150-185 °C, while often being weaker compared to traditionally processed materials. Recently, higher temperature thermoplastics have been manufactured into 3D parts by high temperature SLS that requires a melting temperature of 380 °C, but the usable temperature range for these parts is still under 200 °C.
NASA's thermoset polyimide composites are melt-processable between 150-240 °C, allowing the use of regular SLS machines. The resultant parts are subsequently post-cured using multi-step cycles that slowly heat the material to slightly below its glass transition temperature, while avoiding dimensional change during the process. This invention will greatly benefit aerospace companies in the production of parts with complex geometry for engine components requiring over 300 °C applications, while having a wealth of other potential applications including, but not limited to, printing legacy parts for military aircraft and producing components for high performance electric cars.

Aerofoam
The Aerofoam composites have superior thermal and acoustic insulation properties when compared to conventional polyimide foams. In addition, they provide greater structural integrity than the fragile aerogel materials can provide independently. In general, polymer foams can provide excellent thermal insulation, and polyimide foams have the additional advantage of excellent high-temperature behavior and flame resistance compared to other polymer systems (they do not burn or release noxious chemicals). Incorporating aerogel material into the polyimide foam as described by this technology creates a composite that has been demonstrated to provide additional performance gains, including 25% lower thermal conductivity with no compromise of the structural integrity and high-temperature behavior of the base polyimide foam. The structural properties of Aerofoam are variable based on its
formulation, and it can be used in numerous rigid and flexible foams of varying densities.
Aerofoam has a number of potential commercial applications, including construction, consumer appliances, transportation, electronics, healthcare, and industrial equipment. In addition, these high-performance materials may prove useful in applications that require insulation that can withstand harsh environments, including process piping, tanks for transporting and storing hot or cold fluids, ship and boat building, and aerospace applications.

Blocking/Deblocking Resin Systems
This technology enables the fabrication of co-cured structures without the need for an autoclave or oven large enough to contain the full-scale structure. Instead, sub-components can be prepared in a less expensive, smaller autoclave or oven with co-cure plys applied to the faying surfaces. A continuous, assembled structure can be prepared using a subsequent curing process in a heated press. The co-cured structures can be designed to meet FAA certification criteria for composite structures because no adhesive bond or mechanical fasteners are needed. The structure can be treated as a single, joint-free component.