Projected Background-Oriented Schlieren Imaging
instrumentation
Projected Background-Oriented Schlieren Imaging (LAR-TOPS-365)
Two-camera setup enables real-time, reference-free flow visualization
Overview
Inventors at the NASA Langley Research Center (LAR) have developed a new background-oriented schlieren (BOS) imaging technique. The new technique enables vibration-tolerant, real-time, and reference-free BOS imaging for scientific investigations or industrial flow monitoring. Typically, BOS imaging requires a physical pattern on a retroreflective surface within the flow of interest to visualize that flow through changes in the pattern due to density changes between the pattern and a camera. Here, the BOS imaging technique does not require a physical pattern within the flow, and it is instead projected through the flow onto a retroreflective surface that is then imaged by a camera. Projecting the pattern allows the pattern to be changed mid-measurement and may lessen the cost of BOS imaging for a variety of flow visualization applications.
The Technology
The Projected BOS imaging system developed at the NASA Langley Research Center provides a significant advancement over other BOS flow visualization techniques. Specifically, the present BOS imaging method removes the need for a physically patterned retroreflective background within the flow of interest and is therefore insensitive to the changing conditions due to the flow. For example, in a wind tunnel used for aerodynamics testing, there are vibrations and temperature changes that can affect the entire tunnel and anything inside it. Any patterned background within the wind tunnel will be subject to these changing conditions and those effects must be accounted for in the post-processing of the BOS image. This post-processing is not necessary in the Projected BOS process here.
In the Projected BOS system, a pattern is projected onto a retroreflective background across the flow of interest (Figure 1). The imaged pattern in this configuration can be made physically (a pattern on a transparent slide) or can be digitally produced on an LCD screen. In this projection scheme, a reference image can be taken at the same time as the signal image, facilitating real-time BOS imaging and the pattern to be changed or optimized during the measurements. Thus far, the Projected BOS imaging technology has been proven to work by visualizing the air flow out of a compressed air canister taken with this new system (Figure 2).
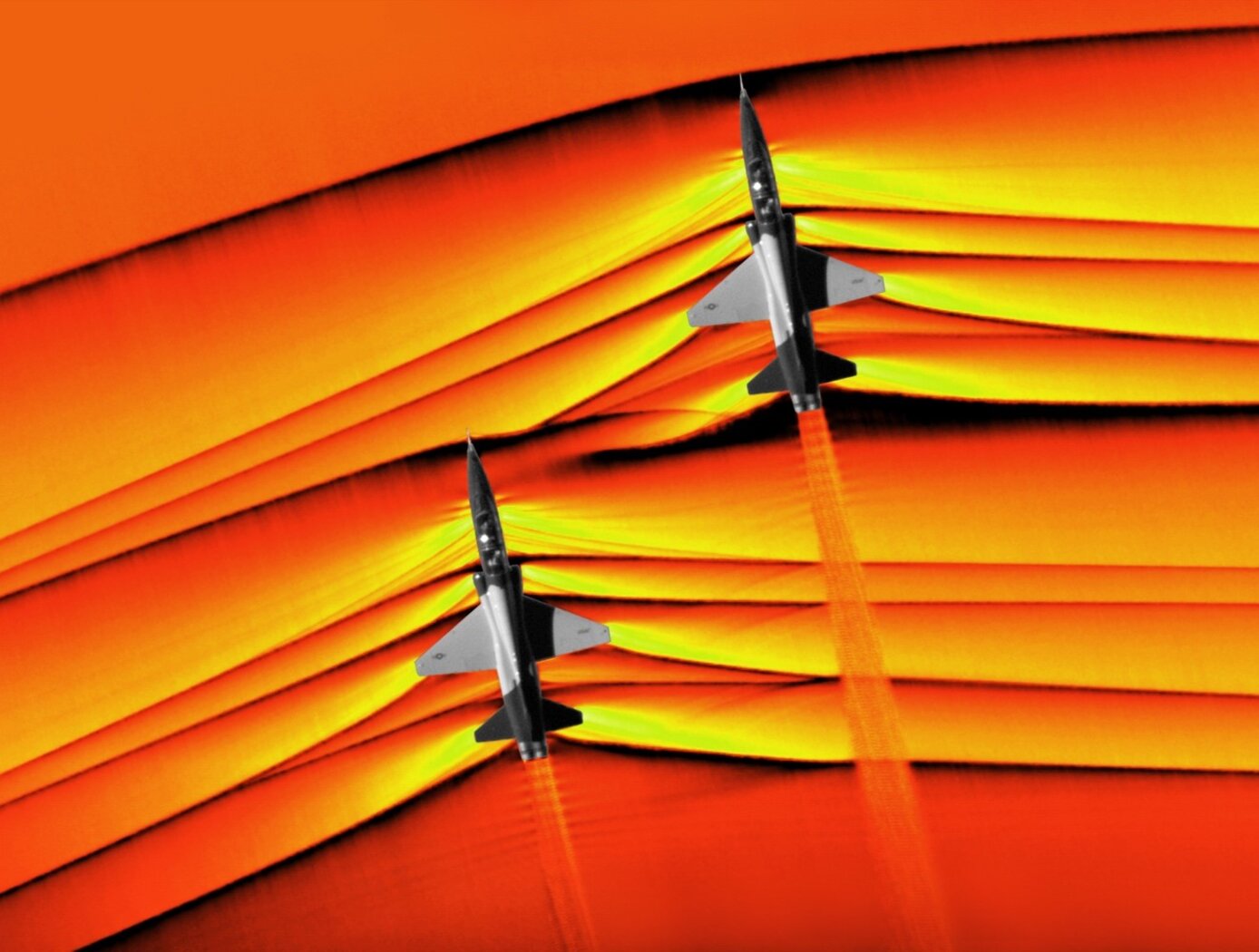
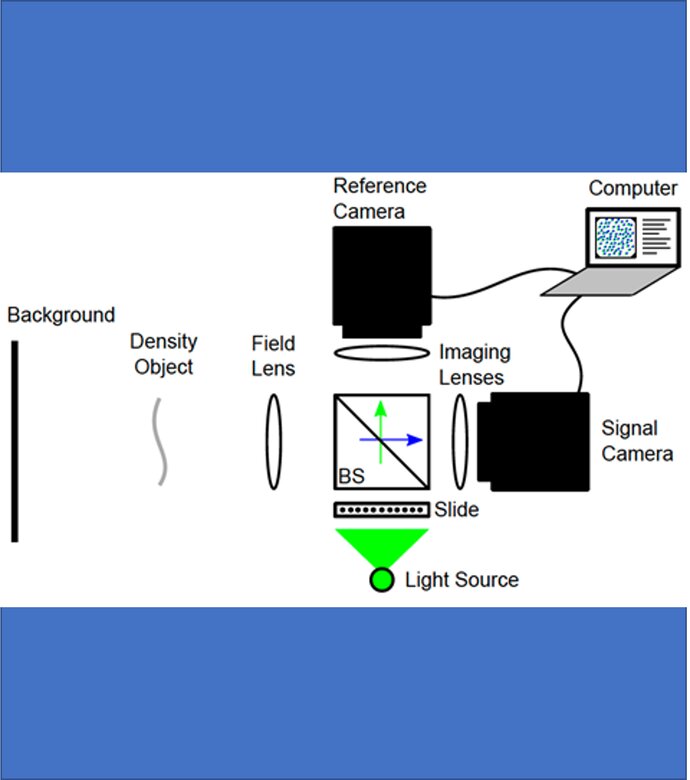
Benefits
- Customizable: the pattern used for imaging can be easily changed during measurements.
- Cheaper: without expensive-to-produce physically patterned retroreflective backgrounds, the flow visualization measurements can be done with less resources.
- Vibration tolerant: without a physical pattern within the flow of interest, the pattern is not affected by vibrations or other changing conditions caused by the flow.
- Real-time measurements: having an included reference camera allows for reference and signal images to be taken simultaneously.
- Multi-functional: the system can be used to do shadowgraph imaging (no pattern needed) in addition to the typical BOS technique.
Applications
- Research: air flow and aerodynamics visualization
- Industrial: thermal management or heat-loss visualization
- Industrial: gas or leak detection
Tags:
|
Similar Results

Assembly for Simplified Hi-Res Flow Visualization
NASAs single grid, self-aligned focusing schlieren optical assembly is attached to a commercial-off-the-shelf camera. It directs light from the light source through a condenser lens and linear polarizer towards a polarizing beam-splitter where the linear, vertically-polarized component of light is reflected onto the optical axis of the instrument. The light passes through a Ronchi ruling grid, a polarizing prism, and a quarter-wave plate prior to projection from the assembly as right-circularly polarized light. The grid-patterned light (having passed through the Ronchi grid) is directed past the density object onto a retroreflective background that serves as the source grid. Upon reflection off the retroreflective background, the polarization state of light is mirrored. It passes the density object a second time and is then reimaged by the system. Upon encountering the polarizing prism the second time, the light is refracted resulting in a slight offset. This refracted light passes through the Ronchi ruling grid, now serving as the cutoff grid, for a second time before being imaged by the camera.
Both small- and large-scale experimental set ups have been evaluated and shown to be capable of fields-of-view of 10 and 300 millimeters respectively. Observed depths of field were found to be comparable to existing systems. Light sources, polarizing prisms, retroreflective materials and lenses can be customized to suit a particular experiment. For example, with a high speed camera and laser light source, the system has collected flow images at a rate of 1MHz.

Reflection-Reducing Imaging System for Machine Vision Applications
NASAs imaging system is comprised of a small CMOS camera fitted with a C-mount lens affixed to a 3D-printed mount. Light from the high-intensity LED is passed through a lens that both diffuses and collimates the LED output, and this light is coupled onto the cameras optical axis using a 50:50 beam-splitting prism.
Use of the collimating/diffusing lens to condition the LED output provides for an illumination source that is of similar diameter to the cameras imaging lens. This is the feature that reduces or eliminates shadows that would otherwise be projected onto the subject plane as a result of refractive index variations in the imaged volume. By coupling the light from the LED unit onto the cameras optical axis, reflections from windows which are often present in wind tunnel facilities to allow for direct views of a test section can be minimized or eliminated when the camera is placed at a small angle of incidence relative to the windows surface. This effect is demonstrated in the image on the bottom left of the page.
Eight imaging systems were fabricated and used for capturing background oriented schlieren (BOS) measurements of flow from a heat gun in the 11-by-11-foot test section of the NASA Ames Unitary Plan Wind Tunnel (see test setup on right). Two additional camera systems (not pictured) captured photogrammetry measurements.

Digital Projection Focusing Schlieren System
NASAs digital projection focusing schlieren system is attached to a commercial-off-the-shelf camera. For focusing schlieren measurements, it directs light from the light source through a condenser lens and linear polarizer towards a beam-splitter where linear, vertically-polarized component of light is reflected onto the optical axis of the instrument. The light passes through the patterned LCD element, a polarizing prism, and a quarter-wave plate prior to projection from the assembly as left- or right-circularly polarized light. The grid-patterned light (having passed through the LCD element) is directed past the density object onto a retroreflective background (RBG) that serves as the source grid. Upon reflection off the RBG, the polarization state of light is mirrored. It passes the density object a second time and is then reimaged by the system. Upon encountering the polarizing prism the second time, the light is slightly offset. This refracted light passes through the LCD element, now serving as the cutoff grid, for a second time before being imaged by the camera.
The LCD element can be programmed to display a variety of grid patterns to enable sensitivity to different density gradients. the color properties of the LCD can be leveraged in combination with multiple colored light sources to enable simultaneous multi-color, multi-technique data collection.
.jpg)
Airborne Background Oriented Schlieren Technique
This invention is an imaging method that requires very simple optics on an airborne vehicle, a camera with an appropriate lens, and an area on the ground that provides visual texture. The complexity with this method is in the image processing and not as much with the hardware or positioning, making Background Oriented Schlieren (BOS) an attractive candidate for obtaining high spatial resolution imaging of shock waves and vortices in flight. First, images are obtained of a visually textured background pattern from an appropriate altitude. Next, a series of images are collected of a vehicle in flight below the observer vehicle and over the same spot on the ground that serves as a background pattern. Shock waves are deduced from distortions of the background pattern resulting from the change in refractive index due to density gradients. The invention requires special software to create the schlieren images. The schlieren image is a contour plot of a two-dimensional data array of measured distortions, in pixel units. The results are used by researchers to help understand the flow phenomenon and compare to computational models. The BOS method also yields measured deflection distances, which can be used to determine the strength of a given density gradient. The system design and flight planning were based on the camera characteristics, airplane coordination, and airspace limitations.

Calculation of Unsteady Aerodynamic Loads Using Fast-Response Pressure-Sensitive Paint (PSP)
Traditionally, unsteady pressure transducers have been the instrumentation of choice for investigating unsteady flow phenomena which can be time-consuming and expensive. The ability to measure and compute these flows has been a long-term challenge for aerospace vehicle designers and manufacturers. Results using only the pressure transducers are prone to inaccuracies, providing overly conservative load predictions in some cases and underestimating load predictions in other areas depending on the flow characteristics. NASA Ames has developed a new state-of-the-art method for measuring fluctuating aerodynamic-induced pressures on wind tunnel models using unsteady Pressure Sensitive Paint (uPSP). The technology couples recent advances in high-speed cameras, high-powered energy sources, and fast response pressure-sensitive paint. The unsteady pressure-sensitive paint (uPSP) technique has emerged as a powerful tool to measure flow, enabling time-resolved measurements of unsteady pressure fluctuations within a dense grid of spatial points on a wind tunnel model. The invention includes details surrounding uPSP processing. This technique enables time-resolved measurements of unsteady pressure fluctuations within a dense grid of spatial points representing the wind tunnel model. Since uPSP is applied by a spray gun, it is continuously distributed. With this approach, if the model geometry can be painted, viewed from a camera, and excited by a lamp source, uPSP data can be collected. Unsteady PSP (uPSP) has the ability to determine more accurate integrated unsteady loads.