Airborne Background Oriented Schlieren Technique
aerospace
Airborne Background Oriented Schlieren Technique (TOP2-271)
Patent Only, No Software Available For License.
Overview
NASA has developed a novel method to render visible the density changes in air that cause a refractive index change by an airborne vehicle. These density changes include shock waves, vortices, engine exhaust, and wakes. The determination of location and strength of shockwaves and vortices is fundamental to understanding the flow around an aircraft. These features are strong enough to affect the environment that the vehicle inhabits: for example, they can cause drag and/or produce undesirable noise. The researcher must be able to predict and mitigate the effects of these flow features. This invention is a robust visualization technique that will permit the measurement of the strengths and positions of shock waves caused by supersonic vehicles. The technique is applicable to all flight regimes, however, and can be used for visualizing tip vortices, engine exhaust plumes.
The Technology
This invention is an imaging method that requires very simple optics on an airborne vehicle, a camera with an appropriate lens, and an area on the ground that provides visual texture. The complexity with this method is in the image processing and not as much with the hardware or positioning, making Background Oriented Schlieren (BOS) an attractive candidate for obtaining high spatial resolution imaging of shock waves and vortices in flight. First, images are obtained of a visually textured background pattern from an appropriate altitude. Next, a series of images are collected of a vehicle in flight below the observer vehicle and over the same spot on the ground that serves as a background pattern. Shock waves are deduced from distortions of the background pattern resulting from the change in refractive index due to density gradients. The invention requires special software to create the schlieren images. The schlieren image is a contour plot of a two-dimensional data array of measured distortions, in pixel units. The results are used by researchers to help understand the flow phenomenon and compare to computational models. The BOS method also yields measured deflection distances, which can be used to determine the strength of a given density gradient. The system design and flight planning were based on the camera characteristics, airplane coordination, and airspace limitations.
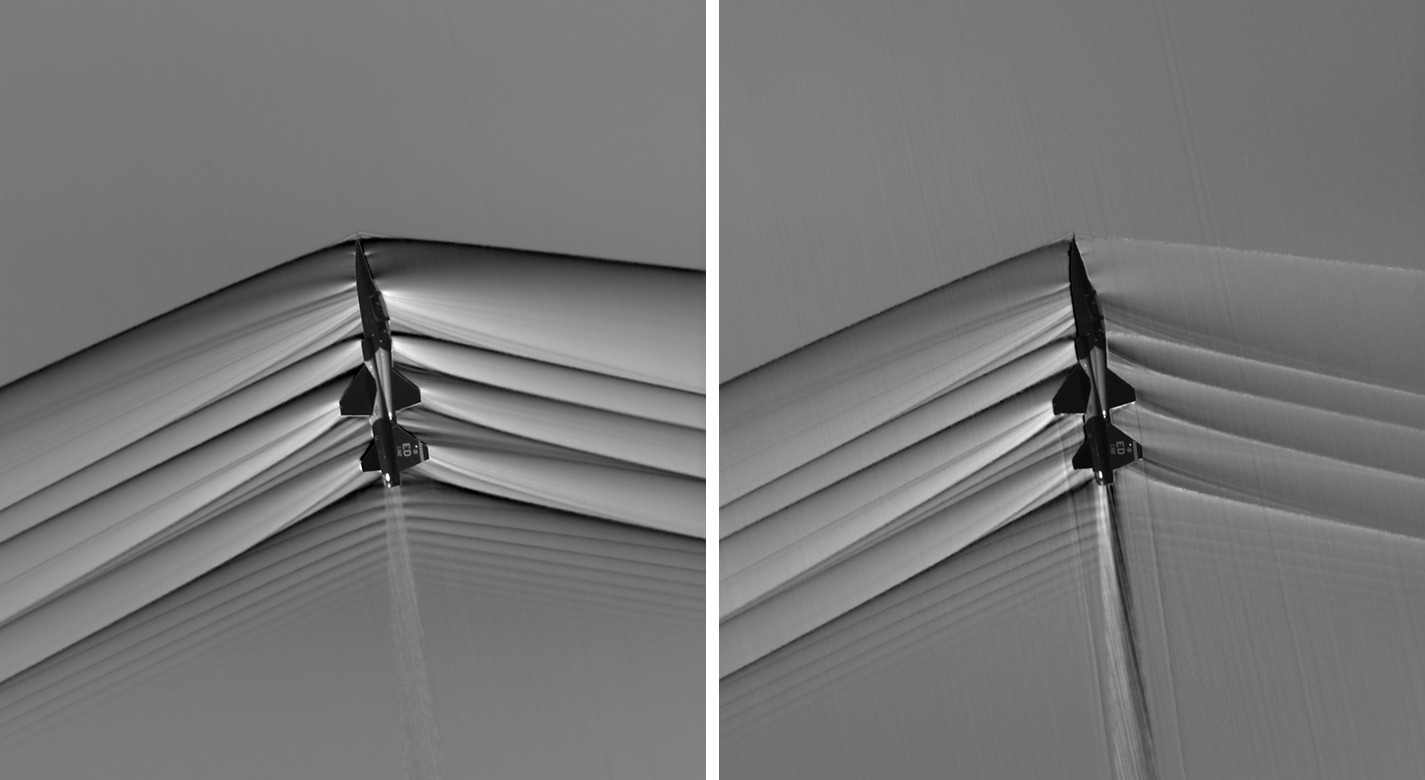
Benefits
- Capture more density gradients details at higher magnifications than other methods
- Gradients can be mapped in any orientation
- The software registers the data image to the reference image which makes the technique robust in off-nominal conditions
- Tracks the movement of the target aircraft to permit averaging and direct velocity measurement
- Requires very simple optics and cameras on an inexpensive air platform
Applications
- Aerospace Industry
- Vortices tracking around airport
- Validate design models of future prototype and demonstrator low-boom aircraft
Similar Results

Projected Background-Oriented Schlieren Imaging
The Projected BOS imaging system developed at the NASA Langley Research Center provides a significant advancement over other BOS flow visualization techniques. Specifically, the present BOS imaging method removes the need for a physically patterned retroreflective background within the flow of interest and is therefore insensitive to the changing conditions due to the flow. For example, in a wind tunnel used for aerodynamics testing, there are vibrations and temperature changes that can affect the entire tunnel and anything inside it. Any patterned background within the wind tunnel will be subject to these changing conditions and those effects must be accounted for in the post-processing of the BOS image. This post-processing is not necessary in the Projected BOS process here.
In the Projected BOS system, a pattern is projected onto a retroreflective background across the flow of interest (Figure 1). The imaged pattern in this configuration can be made physically (a pattern on a transparent slide) or can be digitally produced on an LCD screen. In this projection scheme, a reference image can be taken at the same time as the signal image, facilitating real-time BOS imaging and the pattern to be changed or optimized during the measurements. Thus far, the Projected BOS imaging technology has been proven to work by visualizing the air flow out of a compressed air canister taken with this new system (Figure 2).

Generation of Polystyrene Latex Spheres with Incorporated Fluorescent Dyes
Although polystyrene microspheres are often the seed material of choice for subsonic airflow studies. These seed materials, however, do not provide any benefit for near wall measurements compared to other state-of-the-art seed materials. Consequently, in this innovation NASA scientists have developed this method of generating dye-doped polystyrene microspheres using novel synthetic approaches.
The novel features of this invention are the utility of specific chemical functionalities, monomeric species, environmental additives (buffers), and polyelectrolytes to promote incorporation of dye molecules into developing polystyrene microspheres while enabling control of the spectral properties of the dye relative to pH dependence. These particles will have great utility for wind tunnel measurements near the wall where the state-of-the-art seed materials are not able to collect data. Additionally, the incorporation of these dyes will offer other avenues of data collection including temperature and pressure of the airflows and wind tunnel regions. Likewise, the ability to selectively filter the data collected from these dye-doped polystyrene microspheres can have further applications including the direct visualization of 2 or more fluid flows mixing, among other applications.

Low Frequency Portable Acoustic Measurement System
Langley has developed various technologies to enable the portable detection system, including:
- 3-inch electret condenser microphone - unprecedented sensitivity of -45 dB/Hz
- compact nonporous windscreen - suitable for replacing spatially demanding soaker hoses in current use
- infrasonic calibrator for field use - piston phone with a test signal of 110 dB at 14Hz.
- laboratory calibration apparatus - to very low frequencies
- vacuum isolation vessel - sufficiently anechoic to permit measurement of background noise in microphones at frequencies down to a few Hz
- mobile source for reference - a Helmholtz resonator that provides pure tone at 19 Hz
The NASA system uses a three-element array in the field to locate sources of infrasound and their direction. This information has been correlated with PIREPs available in real time via the Internet, with 10 examples of good correlation.

Calculation of Unsteady Aerodynamic Loads Using Fast-Response Pressure-Sensitive Paint (PSP)
Traditionally, unsteady pressure transducers have been the instrumentation of choice for investigating unsteady flow phenomena which can be time-consuming and expensive. The ability to measure and compute these flows has been a long-term challenge for aerospace vehicle designers and manufacturers. Results using only the pressure transducers are prone to inaccuracies, providing overly conservative load predictions in some cases and underestimating load predictions in other areas depending on the flow characteristics. NASA Ames has developed a new state-of-the-art method for measuring fluctuating aerodynamic-induced pressures on wind tunnel models using unsteady Pressure Sensitive Paint (uPSP). The technology couples recent advances in high-speed cameras, high-powered energy sources, and fast response pressure-sensitive paint. The unsteady pressure-sensitive paint (uPSP) technique has emerged as a powerful tool to measure flow, enabling time-resolved measurements of unsteady pressure fluctuations within a dense grid of spatial points on a wind tunnel model. The invention includes details surrounding uPSP processing. This technique enables time-resolved measurements of unsteady pressure fluctuations within a dense grid of spatial points representing the wind tunnel model. Since uPSP is applied by a spray gun, it is continuously distributed. With this approach, if the model geometry can be painted, viewed from a camera, and excited by a lamp source, uPSP data can be collected. Unsteady PSP (uPSP) has the ability to determine more accurate integrated unsteady loads.

Rapid Aero Modeling for Computational Experiments
RAM-C interfaces with computational software to provide test logic and manage a unique process that implements three main bodies of theory: (a) aircraft system identification (SID), (b) design of experiment (DOE), and (c) CFD. SID defines any number of alternative estimation methods that can be used effectively under the RAM-C process (e.g., machine learning techniques, regression, neural nets, fuzzy modeling, etc.). DOE provides a statistically rigorous, sequential approach that defines the test points required for a given model complexity. Typical DOE test points are optimized to reduce either estimation error or prediction error. CFD provides a large range of fidelity for estimating aircraft aerodynamic responses. In initial implementations, NASA researchers “wrapped” RAM-C around OVERFLOW, a NASA-developed high-fidelity CFD flow solver. Alternative computational software requiring less time and computational resources could be also utilized.
RAM-C generates reduced-order aerodynamic models of aircraft. The software process begins with the user entering a desired level of fidelity and a test configuration defined in terms appropriate for the computational code in use. One can think of the computational code (e.g., high-fidelity CFD flow solver) as the “test facility” with which RAM-C communicates with to guide the modeling process. RAM-C logic determines where data needs to be collected, when the mathematical model structure needs to increase in order, and when the models satisfy the desired level of fidelity.
RAM-C is an efficient, statistically rigorous, automated testing process that only collects data required to identify models that achieve user-defined levels of fidelity – streamlining the modeling process and saving computational resources and time. At NASA, the same Rapid Aero Modeling (RAM) concept has also been applied to other “test facilities” (e.g., wind tunnel test facilities in lieu of CFD software).