How to Train Shape Memory Alloys
materials and coatings
How to Train Shape Memory Alloys (LEW-TOPS-32)
Low cost, time-saving technique to prepare shape memory alloys for use
Overview
Shape memory alloys (SMAs), sometimes known as "smart metals," provide a lightweight, solid-state alternative to conventional actuators and switches, such as hydraulic, pneumatic, or motor-based systems. To function properly, SMAs must be "trained" to return to a previous form when heated, and innovators at NASA's Glenn Research Center have developed a remarkable new method of completing this training at a fraction of the time and cost of conventional training techniques. Glenn's technique uses mechanical cycling, rather than more complicated and time-consuming thermal cycling, to train SMAs before implementation. In addition, this new approach to training allows SMAs to be applied to complex geometric components, so that they may be used in a broader number of applications.
The Technology
Glenn researchers have optimized how shape memory alloys (SMAs) are trained by reconceptualizing the entire stabilization process. Whereas prior techniques stabilize SMAs during thermal cycling, under conditions of fixed stress (known as the isobaric response), what Glenn's innovators have done instead is to use mechanical cycling under conditions of fixed temperature (the isothermal response) to achieve stabilization rapidly and efficiently. This novel method uses the isobaric response to establish the stabilization point under conditions identical to those that will be used during service. Once the stabilization point is known, a set of isothermal mechanical cycling experiments is then performed using different levels of applied stress. Each of these mechanical cycling experiments is left to run until the strain response has stabilized. When the stress levels required to achieve stabilization under isothermal conditions are known, they can be used to train the material in a fraction of the time that would be required to train the material using only thermal cycling. As the strain state has been achieved isothermally, the material can be switched back under isobaric conditions, and will remain stabilized during service. In short, Glenn's method of training can be completed in a matter of minutes rather than in days or even weeks, and so SMAs become much more practical to use in a wide range of applications.
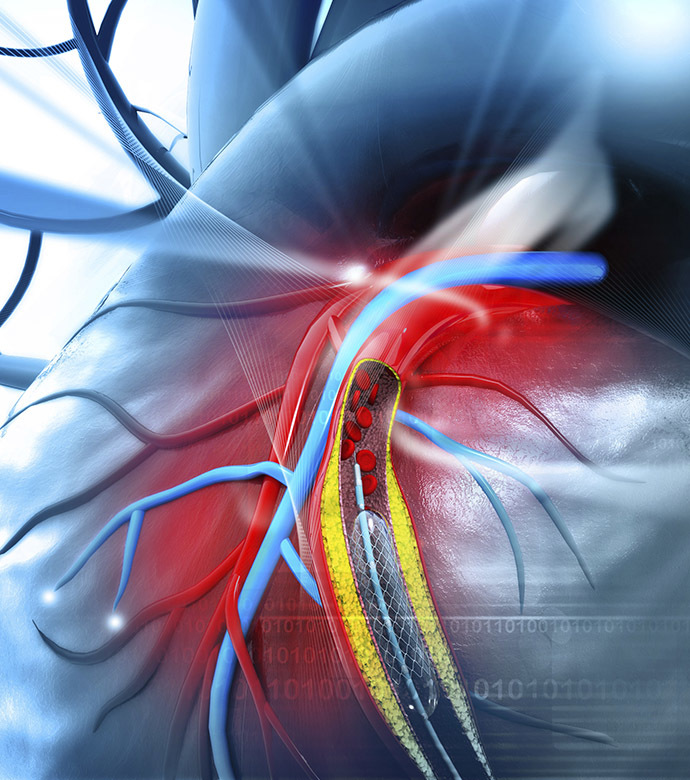
Benefits
- Fast: Stabilizes SMAs in just minutes rather than days or weeks
- Simple: Achieves dimensional stability without the need for time-consuming and tedious processing
- Adaptable: Works for complex geometry components, not just simple geometries like wires or rods
- Reliable: Operates under a wide variety of environmental conditions
Applications
- Aerospace
- Aviation
- Automotive (actuators, engine mounts and suspension, car frames)
- Medical (e.g., stents/angioplasty, bone repair clamps, robotics actuators and micromanipulators that simulate human movement)
- Household appliances (fasteners, seals, connectors, and clamps)
Similar Results

Precipitation Strengthened
Ni-Ti-Pd Shape Memory Alloys
Shape memory alloys (SMAs) are metals that can return to their original shape following thermal input and are largely used as actuators for various applications across industries including space, aeronautics, automotive, and biomedical. These alloys can require long processing times to stabilize through repeated training cycles and suffer from loss of strength and stability during use. Precipitation strengthening (using heat treatments to grow small nanoscale regions of distinct metal phases within the base alloy) is one way to mitigate these issues.
The NASA inventors have combined a modification of typical NiTi compositions by introducing Palladium (Pd) and small amounts of other metals and specific heat treatments to produce a novel SMA with improved properties. Specifically, the alloy is inherently stable, reducing both the need for extended processing times to stabilize the metal and the possibility of failure during high numbers of actuation cycles. Further, the SMA is specifically designed to have significantly lower hysteresis (the temperature difference between the heating and cooling) than current state of the art SMAs, i.e., at or below 10°C compared to 20°C or above. These properties combine for a SMA with enhanced properties usable across various industries and applications for reliable actuation.
The related patent is now available to license. Please note that NASA does not manufacturer products itself for commercial sale.

Shape Memory Alloy Rock Splitters (SMARS)
Glenn's revolutionary SMARS device is fabricated from nickel-titanium-halfnium (NiTiHf), nickel-titanium-zirconium compositions, or a combination. These compositions contain a secondary, nanometer-sized precipitate phase, which is produced through processes of compositional control and ageing heat treatments. Glenn's novel materials and processes have yielded a SMA composition that produces much higher stresses than other SMAs on the commercial market.
The SMARS device is composed of 1) SMA material as the actuating member; 2) a casing heater placed around the SMA member; 3) a DC or AC power source to provide current through the heater; 4) pointed tips for acute penetration into rock formations; and 5) a hand-press to reset the SMA element after each use. In the rock-splitting process, a hole equal to the diameter of the SMA element is drilled in the portion of the rock where the fracture is desired. Next, the pre-compressed SMA is inserted into the hole, and AC or DC current is applied to energize the devices heaters. Once the heater achieves the critical transformation temperature, the SMA will begin to expand within seconds. Since its expansion is constrained by the rock walls, the SMA will eventually exert up to 1500 MPa of stress, splitting the rock apart. When the current is removed and the heater cools, the SMA material returns to its pre-compressed state. At this point, the material can be recovered, so the process is repeatable after reshaping. The SMA actuating members were also designed to achieve displacement greater than the materials strain output. Glenns SMARS device provides high-powered rock fracturing that is controllable, reliable, and comparatively simple without the use of explosives, hydraulics, or chemicals.

Shape Memory Alloy Art (SMArt)
A prototype device has been developed at Glenn for creating shapes from SMA wire. The apparatus uses material feedstock in spools made of alloys that exhibit the shape memory effect (temperature-induced activation), super elasticity (stress-induced activation), and to some extent, magnetism (magnetically-induced activation). The feedstock (e.g., wire spool) is routed and positioned around a series of modular pins to create a shape outline. Once the desired shape is formed, the wire ends are clipped from the feedstock and secured into a locking mechanism, then connected to a heating circuit (e.g., joule heating, hot plate, heat gun). The programmable prescribed circuit parameters, including current or temperature and training time, are set and confirmed using the apparatus control dials and indicators to ensure safe and accurate operation of the device. Before enabling the circuit, a plastic shield is placed over the modular array to protect the operator. The final product will be a desired shape that can be deformed and recovered numerous times through heat activation.
.jpg)
Shape Memory Alloy Mechanisms for CubeSats
Most spacecraft feature release, retention, and deployment devices as key components, because these devices achieve on-demand configurability of solar panels, probes, antennas, scientific instruments, fairings, etc. Until now, designing and using such devices in small spacecraft has been a challenge, because their mass, volume, and power requirements are significant and can impose design constraints. CubeSats, in particular, often need to deploy several structures (such as solar arrays) simultaneously, which prior-art deployment devices have not been able to manage effectively. Glenn's innovation embeds SMAs within the components so the structures can be retained during launch, then released and deployed in orbit. The release and retention device is controlled by an SMA activated pin puller to disengage the release plate from the hooks holding the solar arrays. Once released, the SMA hinge is passively enabled to the deployed state. When ready on orbit, the mechanism is commanded to release and electrical power is sent to the SMA actuator, releasing the component to its deployed state. The component is deployed to its final position through the use of hinges, which are activated passively with SMA spring strips. The retention and release device and hinge are substantially smaller and lighter than deployment mechanisms have ever been and can deploy simultaneously with great reliability. Having already been successfully deployed on a NASA mission, Glenn's innovation is a game-changing technology for CubeSats and other small satellites.

Novel Shape Memory Composite Substrate
The new SMC substrate has four components: a shape memory polymer separately developed at NASA Langley; a stack of thin-ply carbon fiber sheets; a custom heater and heat spreader between the SMC layers; and integrated sensors (temperature and strain). The shape memory polymer allows the as-fabricated substrate to be programmed into a temporary shape through applied force and internal heating. In the programmed shape, the deformed structure is in a frozen state remaining dormant without external constraints. Upon heating once more, the substrate will return slowly (several to tens of seconds) to the original shape (shown below).
The thin carbon fiber laminate and in situ heating solve three major pitfalls of shape memory polymers: low actuation forces, low stiffness and strength limiting use as structural components, and relatively poor heat transfer. The key benefit of the technology is enabling efficient actuation and control of the structure while being a structural component in the load path. Once the SMC substrate is heated and releases its frozen strain energy to return to its original shape, it cools down and rigidizes into a standard polymer composite part. Entire structures can be fabricated from the SMC or it can be a component in the system used for moving between stowed and deployed states (example on the right). These capabilities enable many uses for the technology in-space and terrestrially.