New Concepts in Film Cooling for Turbine Blades
aerospace
New Concepts in Film Cooling for Turbine Blades (LEW-TOPS-52)
Innovative, durable designs increase blade efficiency
Overview
To run at maximum efficiency, turbine engines often experience temperatures of more than 2000 degrees Kelvin, and these elevated temperatures can lead to compromised integrity and failure of the blades. One method for addressing this problem is film cooling, in which cool air is bled from the compressor, ducted to one or more internal chambers of the turbine blades, and discharged through one or more cooling holes to form cooling jets. As a result of the cooling jets, convective heat transfer to the surface of the turbine blade can be reduced. Innovators at NASA's Glenn Research Center have developed two new ways to improve the effectiveness of film cooling. One involves using a shaped recess to create a counter-rotating vortex pair, splitting the incoming flow to a high-energy and a low-energy stream. The other innovation employs various techniques to mitigate the aerodynamic losses that sometime accompany film cooling. These novel approaches make film cooling a much more efficient and successful means of keeping turbine blades operable.
The Technology
In one of NASA Glenn's innovations, a shaped recess can be formed on a surface associated with fluid flow. Often V-shaped, this shaped recess can be configured to create or induce fluid effects, temperature effects, or shedding effects. For example, the shaped recess can be paired (upstream or downstream) with a cooling channel. The configuration of the shaped recess can mitigate the lift-off or separation of the cooling jets that are produced by the cooling channels, thus keeping the cooling jets trained on turbine blades and enhancing the effectiveness of the film-cooling process. The second innovation produced to improve film cooling addresses problems that occur when high-blowing ratios, such as those that occur during transient operation, threaten to diminish cooling effectiveness by creating jet detachment. To keep the cooling jet attached to the turbine blade, and also to spread the jet in the spanwise direction, NASA Glenn inventors have successfully used cooling holes that reduce loss by blowing in the upstream direction. In addition, fences may be used upstream of the holes to bend the cooling flow back toward the downstream direction to further reduce mixing losses. These two innovations represent a significant leap forward in making film cooling for turbine blades, and therefore the operation of turbine engines, more efficient.
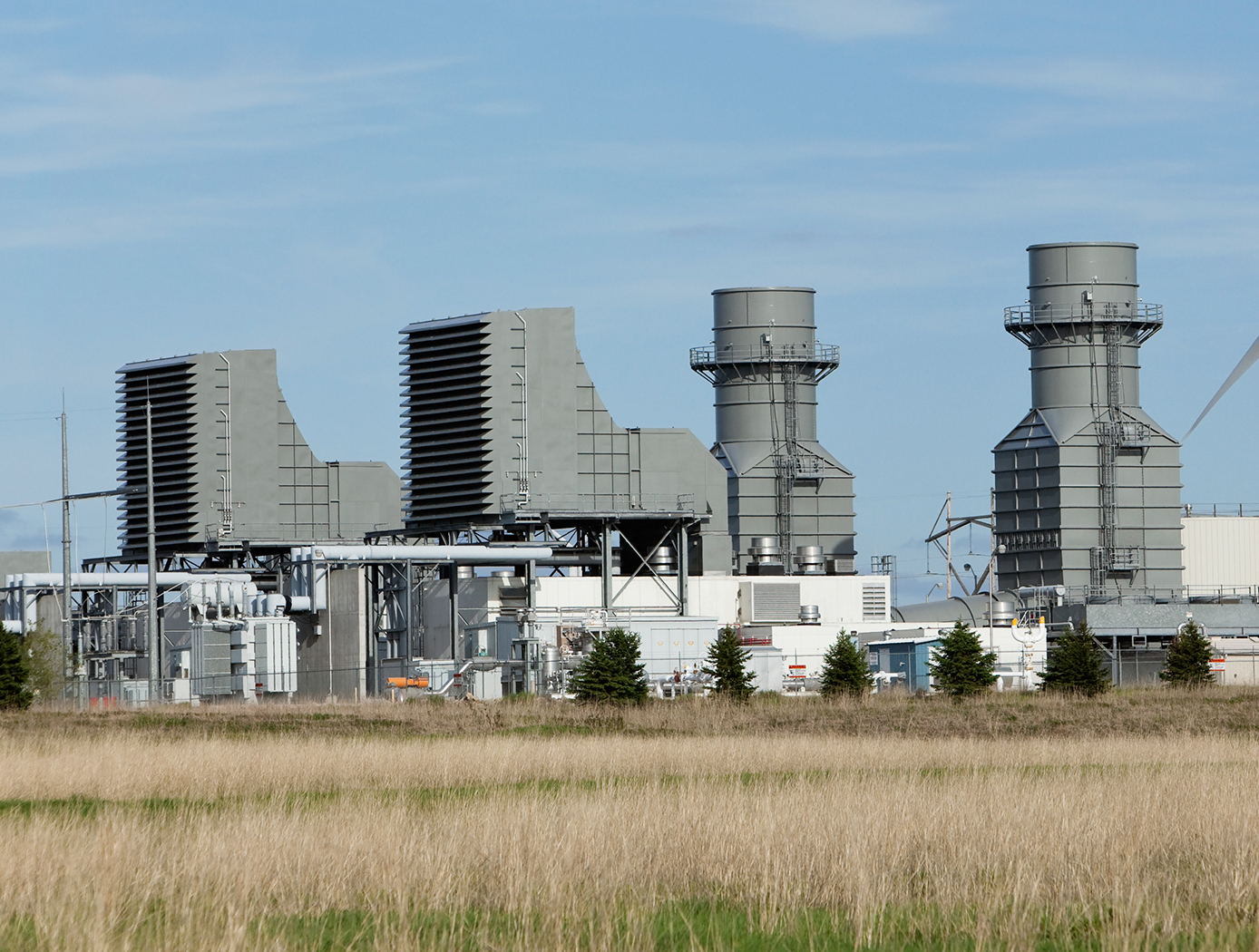
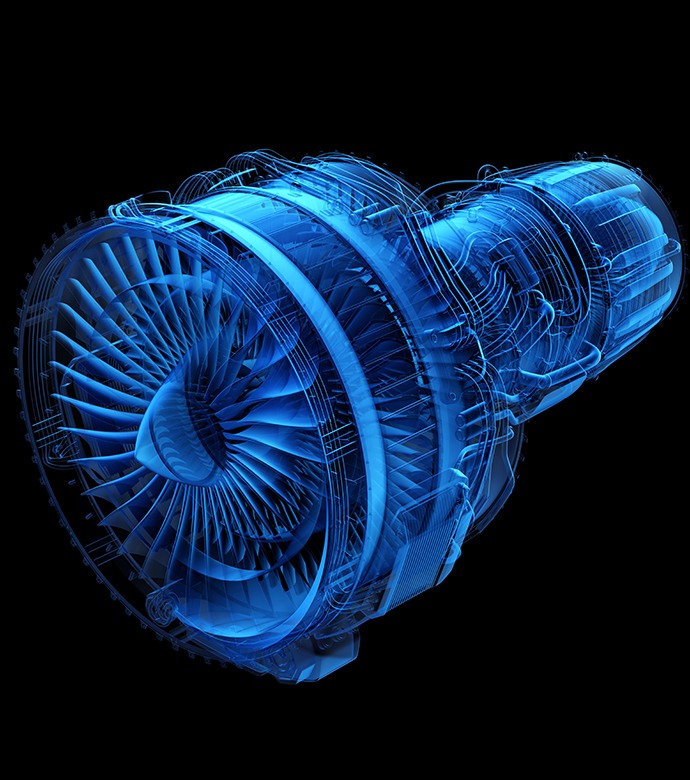
Benefits
- Effective: Reduces aerodynamic losses while still permitting high-temperature operation
- Efficient: Lessens the chance of jet detachment and inefficient cooling
- Clean: Produces more efficient fuel combustion in turbine engines, and burns fuel in a more environmentally friendly way
- Easier to manufacture: Relies on a straightforward design that is simple to fabricate
Applications
- Gas turbines for ground power generation
- Combustion liners
- Jet engines
- High temperature / high pressure gas combustion cooling