Additively Manufactured Propulsion Catalysts
manufacturing
Additively Manufactured Propulsion Catalysts (MFS-TOPS-120)
Ultra-Fine Lattice Structures
Overview
In mono-propulsion systems, a propellant is flowed across a catalyst to promote a chemical reaction and generate thrust. Conventional catalyst manufacturing methods entail (a) coating the inner walls of the thruster nozzle with a catalyst (generally undesirable due to limited catalyst-propellant contact), or (b) inserting a metal or graphite foam coated with a catalyst material into the thruster chamber. Method (b), while superior in terms of thrust generation, also possesses several limitations. Metal/graphite foams are difficult and expensive to procure, stochastic/random in nature (e.g., possess high variability in terms of geometric and other properties), and must be jammed into the thruster chamber using a compression plunger during installation a process that often leads to catalyst damage.
A new manufacturing method that improves repeatability, thruster reactivity, and tailorability (e.g., mechanical, chemical, and fluid flow) while reducing cost and lead time is thus highly desirable. To address this need, NASA and EOS developed methods to additively manufacture (AM) ultra-fine lattice structure propulsion catalysts.
The Technology
Working with EOS, a market leader in additive manufacturing (AM) technology, NASA has pioneered the use of AM to achieve improved performance and cost reduction of propulsion catalyst systems. As mentioned above, conventional mono-propulsion catalysts are comprised of ceramic or graphite foams, which possess thousands of irregular pores with non-uniform size and distribution. Due to the lack of granular control in the manufacturing process, such foams are limited in terms of feasible designs. These foams also exhibit anisotropic mechanical properties and inconsistent fluid flow behavior. Furthermore, such catalysts are produced by a limited number of vendors, constraining availability and inflating cost. As with several other aerospace structures, AM of mono-propulsion catalysts enables increased manufacturing capabilities (e.g., granular geometric control, repeatability) while reducing cost and lead time.
NASA and EOS leveraged AM to generate ultra-fine lattice structures repeating unit cells with ligament thickness as small as 100 microns to replace coated ceramic or graphite foam catalysts. These AM lattice structures offer several advantages including increased control of structure topology, unconstrained designed flexibility, improved compressive strength, and fluid flow optimization all printed into a single component from a preferred refractory platinum metal. Granular control of structure topology allows for tailored percent relative density (%RD), which in turn allows manufacturers to control the mechanical and fluid flow properties of the final catalyst structure. In other words, NASAs AM ultra-fine lattice catalyst structures significantly improve upon the drawbacks of conventional catalysts by offering a higher degree of control over the structure, enabling the generation of catalysts with customized material properties. In addition, the use of AM ensures the catalysts exhibit high spatial symmetry and can be generated in a repeatable (non-stochastic) manner, all while reducing cost and lead times.
While NASA and EOS' ultra-fine lattice structures were originally developed for mono-propellant systems (e.g., green propellant thruster catalysts), the same structures and manufacturing technologies can be applied to liquid or gas permeable rigid materials, evaporative film cooling heat exchangers, filtering, and other applications.
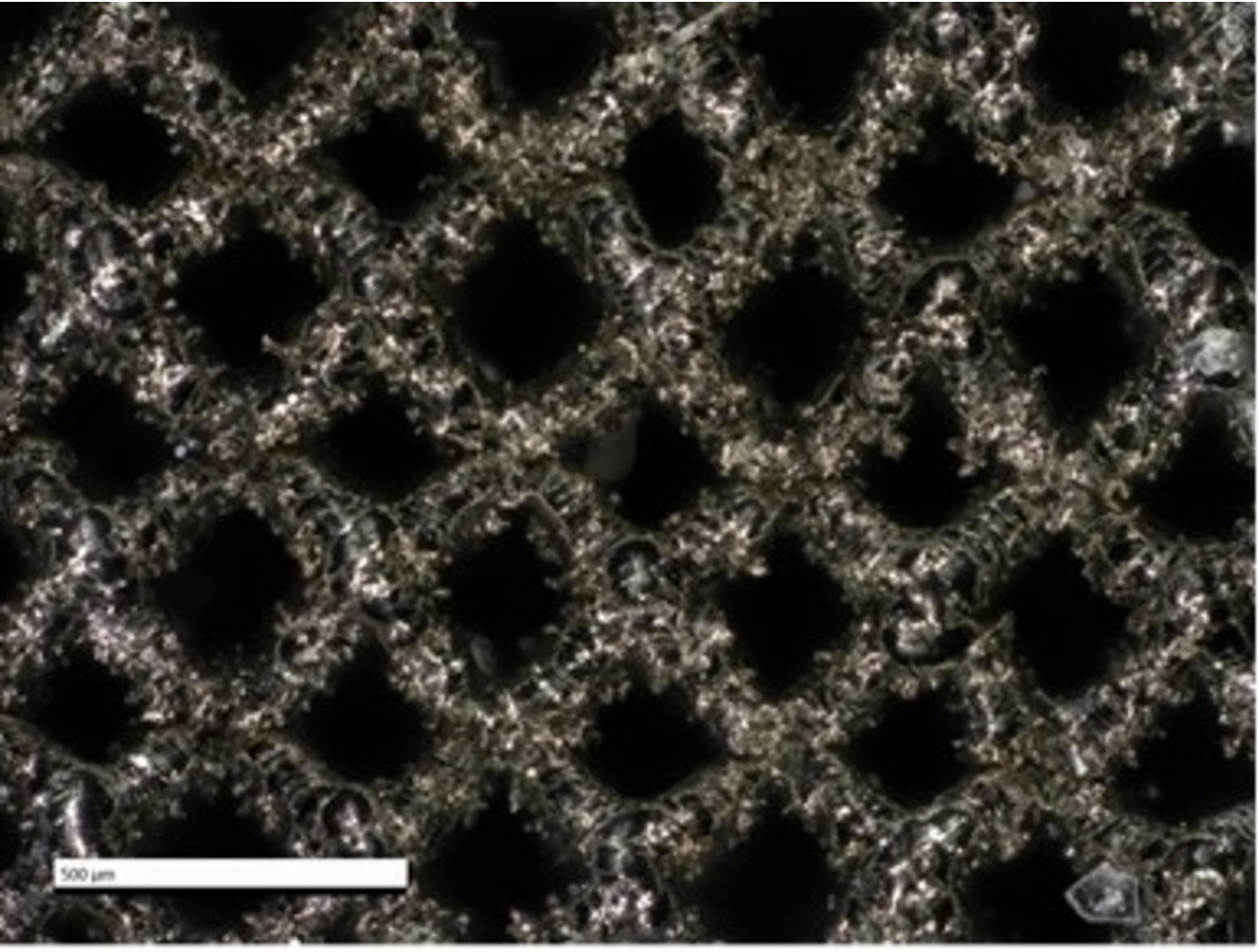
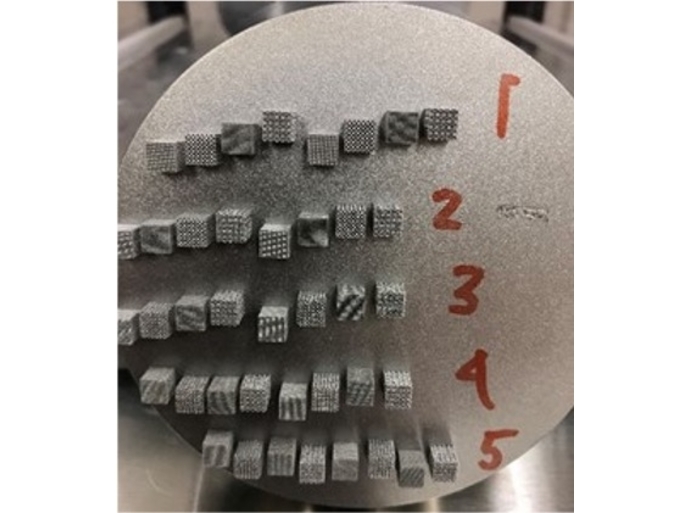
Benefits
- Improved catalyst performance: The ultra-fine lattice structures have been demonstrated to improve compressive strength and flow optimization relative to coated foams.
- Design flexibility: In contrast to coated foams, NASA and EOS' lattice structures offer unconstrained design flexibility - greater control over structure topology allows for customized percent relative density (%RD), which can be manipulated to control mechanical and fluid flow properties.
- Reduced cost & manufacturing schedule: In addition to improved performance, ultra-fine lattice propulsion catalysts can be manufactured at significantly reduced cost, and much faster, relative to coated foam catalysts. Production costs (e.g., feedstock, labor time, post-processing) are very low, with improved schedule control.
- Proven: Testing data demonstrates that ultra-fine lattices meet all the requirements of mono-propellant catalysts and possess mechanical and flow properties as good as, or better than, conventional coated foams.
Applications
- Mono-propellant thruster catalysts: This invention was originally developed to provide thruster catalysts with improved properties for mono-propellant systems (e.g., green propellant thruster catalysts).
- Liquid or gas permeable rigid materials
- Evaporative film cooling heat exchangers
Technology Details
manufacturing
MFS-TOPS-120
MFS-33964-1
"Development of Additive Manufactured Ultra-Fine Lattice Structures Propulsion Catalyst," Mireles, Omar, et. al., August 17, 2020, https://arc.aiaa.org/doi/abs/10.2514/6.2020-3502
Tags:
|
|
Related Links:
|