Diamond Maker Technology Simulates Alien Geology in Laboratories
materials and coatings
Diamond Maker Technology Simulates Alien Geology in Laboratories (MSC-TOPS-89)
Allows researchers ability to speciate natural elements while investigating geologic processes
Overview
Innovators at NASA Johnson Space Center have developed a novel, double capsule control system that allows for high temperature and high-pressure geologic research to be performed in a contained environment relevant to a broad array of materials. It can also yield the speciation of redox-sensitive elements and is even capable of creating geologic conditions necessary to birth diamonds when used in conjunction with a multi-anvil press.
Users of this technology can specify a wide range of oxygen fugacity (fO2) values during experiments. fO2 is a measure of rock oxidation that influences planetary structure and evolution and contributes directly to the study of our galactic origins. It commands some of the fundamental chemical and physical properties in planetary materials, including electrical conductivity, grain-growth kinetics, and phase stability.
This technology was previously used to replicate fO2 environments relevant to core samples from the Moon and those obtained from the Earths deep crust. It may be further extended to higher pressure and higher temperature studies where greater control of a specific experimental sample environment might allow unique chemical bonding and reactivity that would not be possible in systems that utilize the standard approaches.
The Technology
Given the significant impact of fO2 on material properties, it is important to perform studies at fO2 values relevant to the sample of interest. However, current systems used to control fO2 in solid media assemblies (e.g., sliding sensors, graphite capsule buffering) have limited ability to produce the wide fO2 ranges necessary to simulate more extreme planetary interiors.
NASA's fO2 control system implements a modified double capsule design that utilizes a wide range of solid metal-oxide buffers to control fO2 across a wide range of conditions. The approach is a modification of the previous double capsule design wherein the outer capsule itself acts as the metal component of the buffer assemblage, and the inner capsule to which the astromaterial sample of interest is placed resides within the outer capsule.
To achieve higher experimental temperatures above the melting point of more traditional noble metal capsule materials, outer capsules of the control assembly are comprised of refractory (high melting point) metals such as Ni, Co, W, Fe, Mo, V, Cr, Nb and Ta. Resultant experiments have reliably achieved temperatures exceeding 1600 degrees C, and when used in conjunction with a multi-anvil press, system pressures of over 20 GPa can be obtained lending researchers the ability to simulate on or off-world geologic conditions previously difficult to obtain in a laboratory.
NASA's fO2 control system was developed to enable high pressure, high temperature experimental studies of astromaterials at fO2 values relevant to the sample of interest. However, it may also be useful for the synthesis of materials where fO2 control is required (e.g., synthesis of crystal structures that might be stable under higher oxygen pressure). Further use cases may include mineral or melt syntheses, metal-silicate or mineral-melt element partitioning, phase equilibria studies, and the possible development of new chemical and mineral compounds that could not be manufactured in laboratories before.
This fO2 control system technology is at a technology readiness level (TRL) 6 (system/subsystem prototype demonstration in a relevant environment). The innovation is now available for your company to license. Please note that NASA does not manufacture products itself for commercial sale.
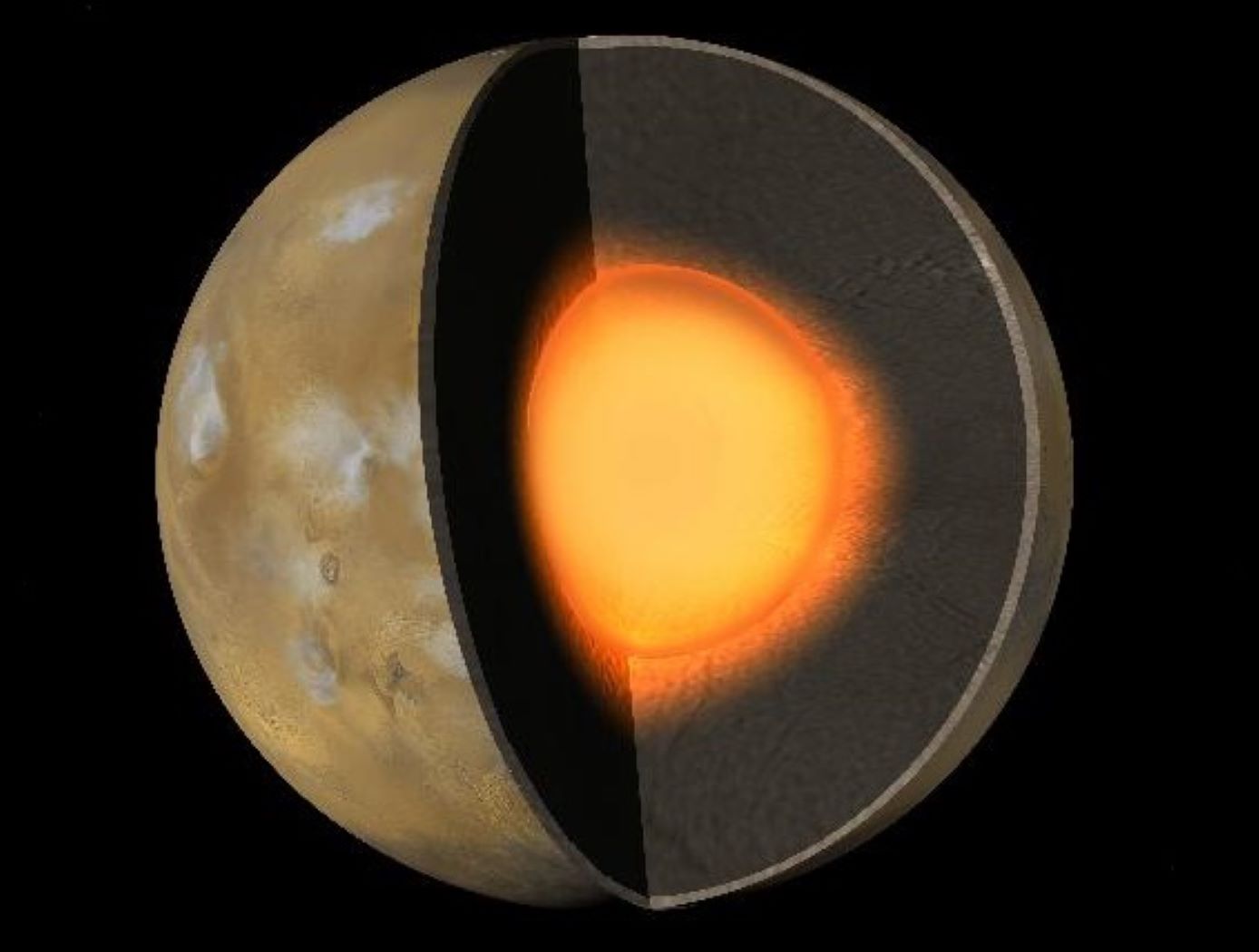
Benefits
- Improves simulation of more extreme planetary interiors by yielding higher temperature (1600+C) and pressure values (20+GPa).
- Allows fO2 to be specified across wider ranges of values relevant to experimental samples.
- Promotes unique chemical bonding and reactivity that may not be possible in other systems.
- Yields speciation of redox-sensitive elements.
- System has been trained on core samples from the Moon and the Earths deep crust.
- Accelerates research into stellar neighborhood and galactic origins.
Applications
- High pressure, high temperature geological studies
- Materials science and engineering
- Melt synthesis
- Metal-silicate or mineral-melt partitioning
- Phase equilibria studies
- Pharmaceutical research
- Synthesis of new mineral and chemical compounds
Tags:
|
|
Related Links:
|
Similar Results

Room temperature oxygen sensors
NASA Ames has developed very small-sized oxygen sensors made of a graphene and titanium dioxide (TiO2) hybrid material. With ultraviolet (UV) illumination, these sensors are capable of detecting oxygen (O2) gas at room temperature and at ambient pressure. The sensors are able to detect oxygen at concentrations ranging from about 0.2% to about 10% by volume under 365nm UV light, and at concentrations ranging from 0.4% to 20% by volume under short wave 254nm UV light. These sensors have fast response and recovery times and can also be used to detect ozone.
This unique room temperature O2 sensor provides significant advantages in O2 sensing applications, especially those applications where high operating temperature requirements cannot be met, or would result in inefficient manufacturing processes. Since graphene is not intrinsically responsive to O2, and TiO2 is not responsive to oxygen at room temperature, the materials are first synthesized as a hybrid material. The synthesized graphene- TiO2 hybrid material is then ultrasonicated and then drop-casted onto a series of Interdigitated Electrodes (IDE) to form the sensors.
Ultrasonication ensures effective charge transfer at the graphene- TiO2 interphase. The graphene and the titanium dioxide may be present in the composite material in different ratios to ensure optimal oxygen detection. It is the combination of graphene with TiO2 that yields a semiconducting material capable of O2 sensing at room-temperature operation.

Modular Artificial-Gravity Orbital Refinery Spacecraft
Modular Artificial-Gravity Orbital Refinery Spacecraft is a solution for refining in-situ materials collected in space, such as from asteroids and Mars moons, as well as recycling spacecraft debris, while orbiting in micro-gravity conditions. The spacecraft is coupled with refining modules for refining and recycling different types of materials. It generates artificial gravity for operation in low-gravity environments. The spacecraft is comprised of rotating rings, each generating artificial gravity and angular momentum. When the rotating rings are combined on the spacecraft platform, however, they have a net near-zero angular momentum such that the spacecraft can change its attitude with minimal propellant or rotate at the rate of the object the spacecraft platform is attached to. The spacecraft platform can self-balance to accommodate different sized modules and modules with moving loads. The refined and recycled materials can be used to create products in-situ as well as products too large to launch from Earth, such as construction of orbiting space habitats, large spacecraft, solar-power stations, and observatories.

Improved Lunar Regolith Simulant Ion Implantation
Researchers and other technology developers require regolith simulants that accurately emulate the properties of lunar, Martian, and asteroid soils to ensure that the processes, devices, tools, and sensors being developed will be usable in an active mission environment. To move toward higher fidelity regolith simulants, NASA has developed a system that takes typical regolith simulants and implants ions of relevant elements to better simulate the conditions of extraterrestrial soils.
The ion implantation device developed here is composed of three key elements as shown in the figure below: two hopper and rotary valve elements and the acceleration grid structure. To perform the ion implantation, the system is first placed within a vacuum chamber, pumped down, and gases of the elements of interest are pumped into the chamber. The system then first passes a mass of granulated lunar regolith simulant through two stages of hoppers and rotary valves to condition the material. Key to the system is a process for interstitial gas removal (a source of contamination) as shown in the figure on the right. After conditioning, the regolith simulant is passed between two parallel electrodes under a high voltage, accelerating ions of the process gas and implanting those ions within the regolith simulant at controllable depths.
The related patent is now available to license. Please note that NASA does not manufacturer products itself for commercial sale.

Carbonated Cement for Production of Concrete with Improved Properties
The NASA cement innovation describes a method to make solid carbon material from CO2 captured during the cement-making process, and for using that carbon material in the mixture to improve cement properties. Doing so provides a direct use for the captured CO2, eliminating any CO2 storage/disposal issues and providing an improved cement product.
The innovation employs a chemical reaction, known as the Bosch process, which uses hydrogen gas and catalysis to reduce the CO2 to solid carbon and water. Cement manufacturing is uniquely suited to the use of the Bosch process. Cement manufacturing requires high temperatures, and harnessing this excess heat limits the total energy required to maintain a Bosch process at a cement plant. Also, cement contains iron, a metal shown to be an exceptional catalyst for the Bosch process. Thus, the cement product itself can be used as the catalyst for the reaction, also serving as a carbon sink. This eliminates any requirements for the storage or disposal of the waste carbon captured from CO2 emissions.
Test evaluations at the bench scale have provided encouraging indications of enhanced mechanical properties for the carbon-containing cement materials. In particular, the findings suggest that the carbon in the concrete might delay the environmental breakdown of concrete due to the blocking effect of the carbon on harmful ions (e.g., chlorine).
Cryogenic Oxygen Storage Modules COSM
The COSM employs NASA's Cryogenic Flux Capacitor core to store liquid oxygen (at 90 K) in silica aerogel material at ambient pressure, and then discharges cold oxygen gas into an in-line flow loop in response to heat input. If the composition of the incoming effluent stream contains gases with condensation or freezing points above the 90 K oxygen storage temperature--such as carbon dioxide or water vapor--these gasses can be removed from the stream as it moves through the COSM. The current COSM is sized to be wearable on the person but can be easily scaled to much larger sizes and various geometries.
COSM is designed with a long "cold path" which provides for greater residence times which increase the probability that condensable/freezable gases will be trapped in the COSM. Also, the longer the cold path, the longer the time a COSM can be used prior to the oxygen being depleted and the scrubbed gasses liberated. Two COSM geometries have been designed, built, and tested-a round spiral and a prismatic serpentine--to achieve long cold paths, and intrinsic vapor cooling to manage heat loads.