In Situ Performance Monitoring of Piezoelectric Sensors and Accelerometers
sensors
In Situ Performance Monitoring of Piezoelectric Sensors and Accelerometers (SSC-TOPS-1)
Tests and Identifies Degraded Sensors Without Having to Remove Them
Overview
An in situ measurement system for monitoring the performance of piezoelectric sensors, particularly accelerometers, was developed. With this technology, characteristics such as resonant frequency, response, cable status, connectivity, bonding and linear range, can be determined. Sensors can be tested in a very wide frequency range, extending to 200 MHz and beyond, without removing them from their mounted locations, and without requiring specially constructed transducers or special wiring. Assessments can be performed in situ, and can be conducted with hand held test equipment or integrated into instrumentation systems. With this monitoring system, degraded sensor performance can be quickly and economically identified with a handheld unit or integrated directly into test equipment.
The Technology
On occasion, anomalies may appear in the highly dynamic test data obtained during rocket engine tests, which are investigated and corrective action may be mandated before subsequent testing. Also, it is often unclear if anomalies in recorded signals are due to differences between the Low and High Speed Data Acquisitions Systems, difference between the transducers, a failed transducer, or if everything is working correctly and the system were actually accurately recording real events. Commercial test equipment suitable for testing piezoelectric sensors is expensive and requires that the sensor be removed from the test article for evaluation. With the monitoring system developed, degraded sensor performance can be quickly and economically identified.
This system can evaluate installed piezoelectric sensors, without requiring physical contact with or removing them from their mounted locations. Tests are conducted through cabling. Since it is not necessary to remove the device, data that reflect the devices specific physical configuration (such as as-mounted resonant frequency) are retained, and devices that are physically inaccessible can still be tested. The testing system is not limited to identifying degraded performance in the sensors piezoelectric elements; it can detect changes within the entire sensor, and sensor housing.
The system can be made portable, in a battery powered sealed box, for testing in the field. Since physical contact with the sensor is not necessary, therefore, monitoring can be done as far away as 250 feet, or longer if certain provisions are made.
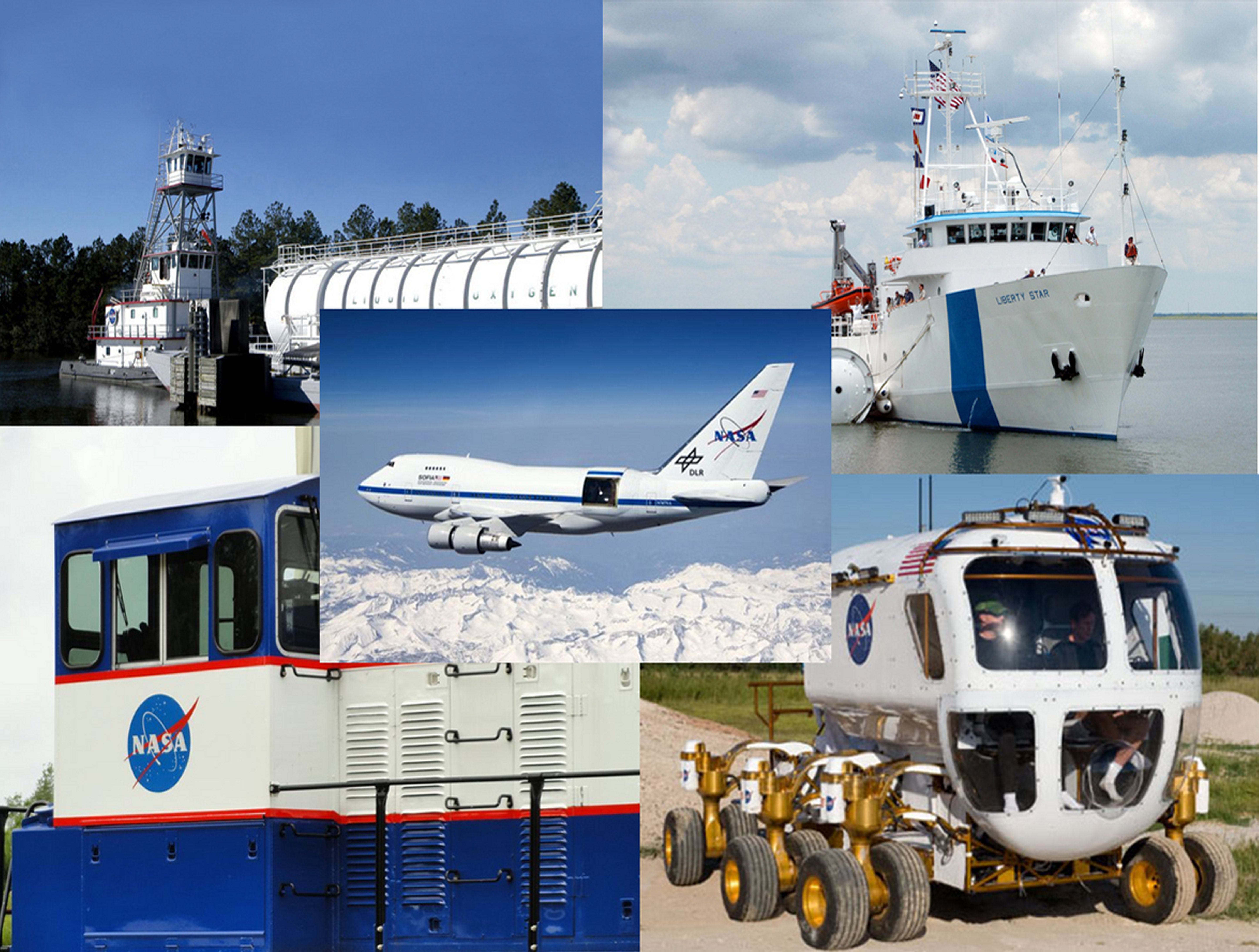
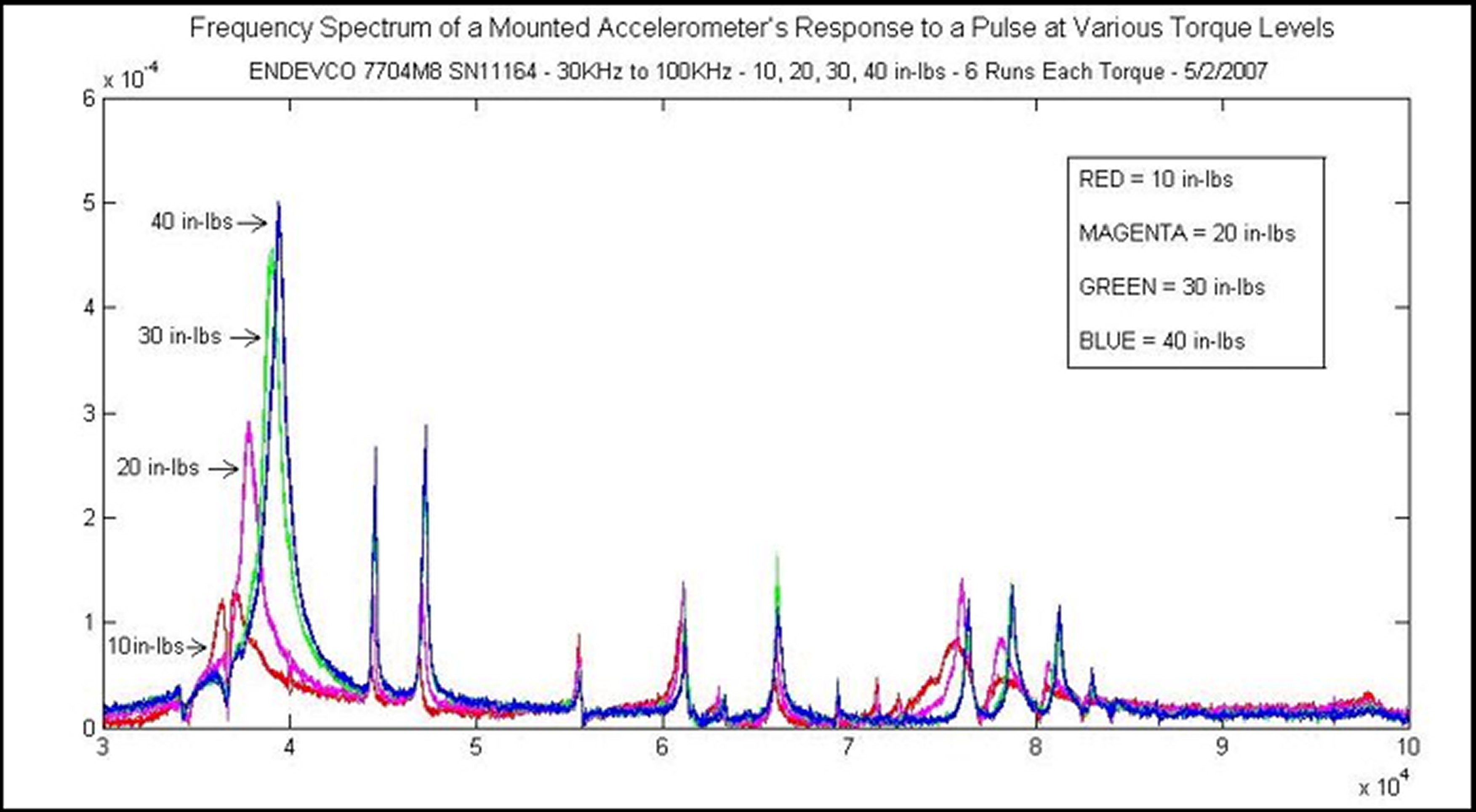
Benefits
- Simplified Testing: Allows testing of piezoelectric sensors without requiring physical contact
- Reduced Re-Calibration Costs: Does not require removing mounted sensor or sending to a calibration lab
- Increased Testing Parameter Range: Provides both normal and as-mounted resonant frequencies
- Improved Accuracy: Provides entire frequency response over the range of the device (unlike commonly used shaker tables)
- Identifies In Situ Problems: Can identify degraded sensor bonds, faulty cabling and sensor damage
Applications
- Accelerometers
- Automotive sensors
- Structural sensors
- Sensors for manufacturing equipment
- Any application where vibration is monitored
- Any piezoelectric sensor
- Applicable in Nondestructive Testing (NDT)
Technology Details
sensors
SSC-TOPS-1
SSC-00327
Jensen, Scott. "In Situ Health Monitoring of Piezoelectric Sensors." TechConnect World Innovation Conference & Expo, June 17, 2015, Washington, DC.
Jensen, Scott. "In Situ Performance Monitoring of Piezoelectric Sensors and Accelerometers." NOBIC (New Orleans BioInnovation Center) Technology Showcase, Innovation Louisiana 2015 Conference, November 18, 2015 New Orleans, LA.
Jensen, Scott. "In Situ Performance Monitoring of Piezoelectric Sensors and Accelerometers." NOBIC (New Orleans BioInnovation Center) Technology Showcase, Innovation Louisiana 2015 Conference, November 18, 2015 New Orleans, LA.
Tags:
|
Similar Results

Relaxor Piezoelectric Single Crystal Multilayer Stacks for Energy Harvesting Transducers (RPSEHT)
Energy management is one of the most challenging issues in the world today. Accordingly, various energy harvesting technologies have gained attention, including harvesting energy from ambient vibration sources using piezoelectric materials. However, conventional piezoelectric energy harvesting transducer (PEHT) structures have effective piezoelectric constants that are lower than about 10^4 pC/N, (resonant mode). These low piezoelectric constants lead to conventional PEHTs not being able to harvest electric power effectively. Further, for a specific vibration/motion source, it would be advantageous to maximize the mechanical energy captured from the vibration structure into the piezoelectric device and to convert a greater fraction of that mechanical energy into electrical energy more efficiently.
This invention is a system and method using multistage force amplification of piezoelectric energy harvesting transducers (MFAPEHTs) to increase the effective piezoelectric constant to >10^6 pC/N and to increase the mechanical energy input to the device. The invention utilizes 33 mode PZT to permit maximum coupling between the input mechanical energy with the piezoelectric material, and multilayer construction of single crystal PMN-PT material to significantly amplify the voltage/charge generation and storage from the applied mechanical force.

Damage and Tamper Detection Sensor System
The SansEC sensor system consists of multiple pairs of inductor-capacitor sensors with no electrical connections, which are placed throughout the material being monitored for damage. The sensors are embedded in or placed directly onto the surface of the material. Strains and breaks are detected by changes in resonant frequency read by the accompanying magnetic field data acquisition system. When pulsed by a sequence of magnetic field harmonics from the acquisition system, the sensors become electrically active and emit a wireless response. The magnetic field response attributes of frequency, amplitude, and bandwidth of the inductor correspond to the physical property states measured by the sensor. The received response is correlated to calibration data to determine the physical property measurement. Because each sensor pair has its own frequency response, when damage occurs to that circuit the frequency response changes. This change identifies the damage location within the material.
A unique feature achieved by eliminating electrical connections is that damage to a single point will not prevent the sensor from being powered or interrogated. If a sensor is broken, two concentric inductively coupled sensors are created, thus identifying tamper or damage location.

Low-Profile Wireless Sensor
The low-profile sensor is configured with a spiral electrical trace on flexible substrate. In typical inductor designs, the space between traces is designed to minimize parasitic conductance to reduce the impact of the capacitance to neighboring electronics. In the low-profile sensor, however, greater capacitance is desired to allow the operation of an inductor-capacitor circuit. This allows the traces to be closer together, decreasing the
overall size of the spiral trace.
The sensor receives a signal from the accompanying magnetic field data acquisition system. Once electrically active, the sensor produces its own harmonic magnetic field as the inductor stores and releases magnetic energy. The antenna of the measurement acquisition system is switched from a transmitting to a receiving mode to acquire the magnetic-field response of the sensor. The magnetic-field response attributes of frequency, amplitude, and bandwidth of the inductor correspond to the physical property states measured by the sensor. The received response is correlated to calibration data to determine the physical property measurement. When multiple sensors are inductively coupled, the data acquisition system needs to activate and read
only one sensor to obtain measurement data from all of them.

Novel Solid-State Humidity Sensor
NASAs novel ceramic dielectric material enables extremely high-sensitivity humidity sensing. The ceramic sensing element is robust, can be manufactured using printing processes, and exhibits fast response and recovery speeds with large capacitance and resistance response/change per relative humidity unit change across a wide range of humidity levels in a log-linear response. Preliminary test data conducted in a humidity test chamber show a log-linear measured response in capacitance from 5 nanofarads (at 30% relative humidity, room temperature) to 0.2 millifarads (at 90% relative humidity, room temperature).
The inventors discovered the humidity sensing element technology during their efforts to develop next-generation energy storage materials and devices for NASA. The inventors were initially puzzled by large swings in capacitance observed over the course of any given day in one particular dielectric composition, and, ultimately, they were able to trace these unexpected changes in capacitance back to corresponding changes in ambient humidity, even those occurring from breathing and exhalation.
The sensor element can be formed using a dielectric ink or paste formulation, also developed by NASA, via traditional screen printing or advanced ink jet, aerosol, or 3D printing methods. The printed sensor element can be very thin, on the order of microns in thickness, with a small footprint, one square centimeter or less.

Fiber Optic Sensing Technologies
The FOSS technology revolutionizes fiber optic sensing by using its innovative algorithms to calculate a range of useful parameters—any and all of which can be monitored simultaneously and in real time. FOSS also couples these cutting-edge algorithms with a high-speed, low-cost processing platform and interrogator to create a single, robust, stand-alone instrumentation system. The system distributes thousands of sensors in a vast network—much like the human body's nervous system—that provides valuable information.
How It Works
Fiber Bragg grating (FBG) sensors are embedded in an optical fiber at intervals as small as 0.25 inches, which is then attached to or integrated into the structure. An innovative, low-cost, temperature-tuned distributed feedback (DFB) laser with no moving parts interrogates the FBG sensors as they respond to changes in optical wavelength resulting from stress or pressure on the structure, sending the data to a processing system. Unique algorithms correlate optical response to displacement data, calculating the shape and movement of the optical fiber (and, by extension, the structure) in real time, without affecting the structure's intrinsic properties. The system uses these data to calculate additional parameters, displaying parameters such as 2D and 3D shape/position, temperature, liquid level, stiffness, strength, pressure, stress, and operational loads.
Why It Is Better
FOSS monitors strain, stresses, structural instabilities, temperature distributions, and a plethora of other engineering measurements in real time with a single instrumentation system weighing less than 10 pounds. FOSS can also discern between liquid and gas states in a tank or other container, providing accurate measurements at 0.25-inch intervals. Adaptive spatial resolution features enable faster signal processing and precision measurement only when and where it is needed, saving time and resources. As a result, FOSS lends itself well to long-term bandwidth-limited monitoring of structures that experience few variations but could be vulnerable as anomalies occur (e.g., a bridge stressed by strong wind gusts or an earthquake).
As a single example of the value FOSS can provide, consider oil and gas drilling applications. The FOSS technology could be incorporated into specialized drill heads to sense drill direction as well as temperature and pressure. Because FOSS accurately determines the drill shape, users can position the drill head exactly as needed. Temperature and pressure indicate the health of the drill. This type of strain and temperature monitoring could also be applied to sophisticated industrial bore scope usage in drilling and exploration.
For more information about the full portfolio of FOSS technologies, see visit https://technology-afrc.ndc.nasa.gov/featurestory/fiber-optic-sensing