Reversible Androgynous Mechanical Fastener
robotics automation and control
Reversible Androgynous Mechanical Fastener (TOP2-310)
Androgynous Fasteners for Robotic Structural Assembly
Overview
Researchers at NASA Ames Research Center have developed an androgynous fastener with high misalignment tolerance characteristics, which is suitable for robotic actuation. This fastener was developed in conjunction with a high-performance building-block structural system that can be robotically assembled by robust collective automated assembly into large, reconfigurable structures ranging from assembly of lunar habitats to terrestrial structures. The fastener mechanisms employ alignment principles similar to the International Berthing and Docking Mechanism (IBDM) in order to relax the positioning requirements of the assembly robots. This novel androgynous fastener provides the desired performance required for robotic assembly of the structural systems and also minimizes or eliminates the problems and disadvantages associated with conventional or traditional fasteners.
The Technology
The androgynous fastener is lightweight and facilitates assembly through simple actuation with large driver-positioning tolerance requirements. This fastener provides a high-strength, reversible mechanical connection and may be used in high strength-to-weight ratio structural systems, such as lattice structure systems. The androgynous fastener resists tensile and shear forces upon loading of the lattice structure system thereby ensuring that the struts of the lattice structure system govern the mechanical behavior of the system. The androgynous fastener eliminates building-block orientation requirements and allows assembly in all orthogonal build directions. This androgynous fastener may be captive in building-block structural elements thereby minimizing the logistical complexity of transporting additional fasteners. Integration of a plurality of the androgynous fasteners into a high performance, robotically managed, structural system reduces launch energy requirements, enables higher mission adaptivity and decreases system life-cycle costs. The androgynous fastener is beneficial in any application where robotic end effectors are used to join structural components (or other parts) together. It may be particularly desirable for applications requiring frequent movement of hardware to an assembly site to replace joint connections.
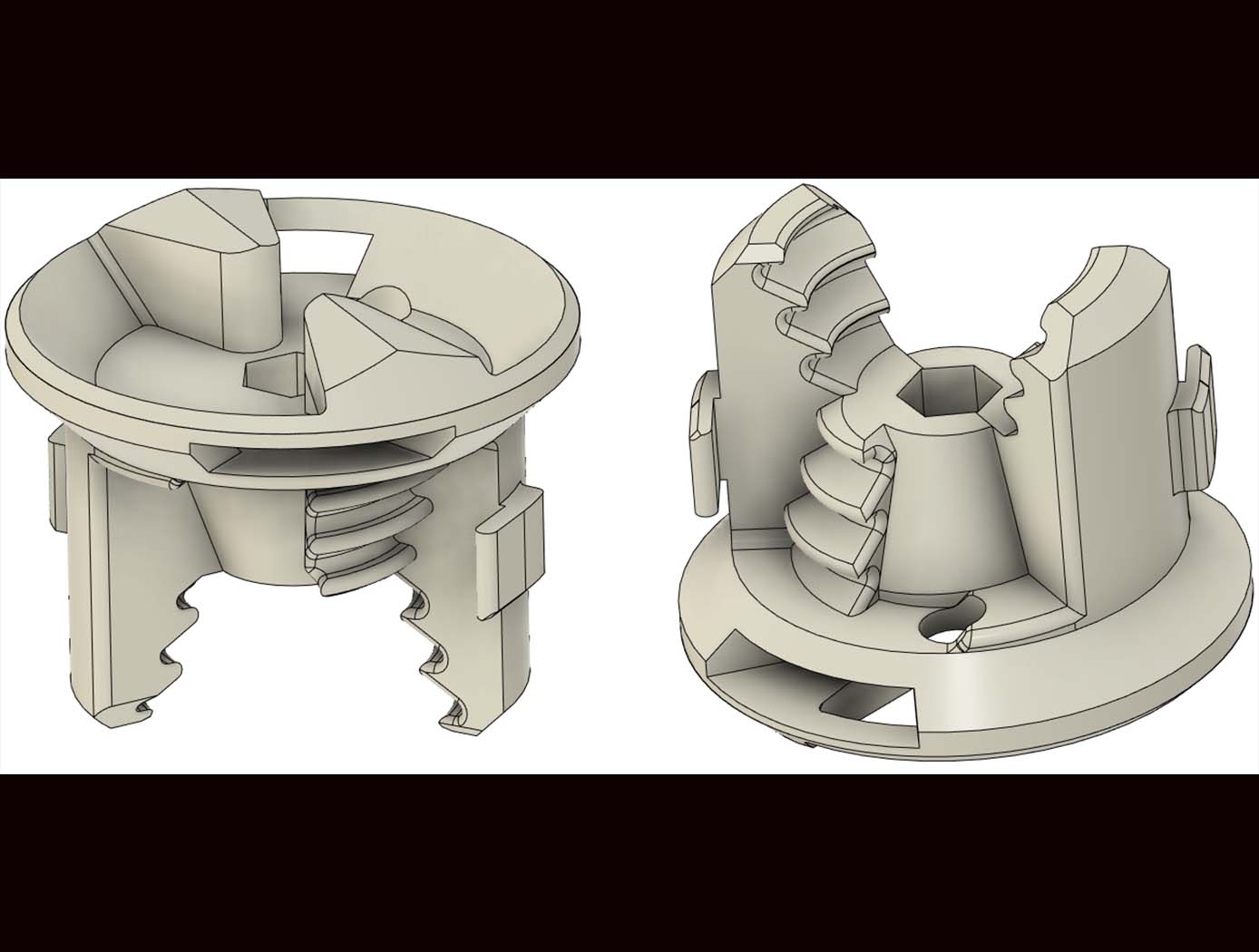
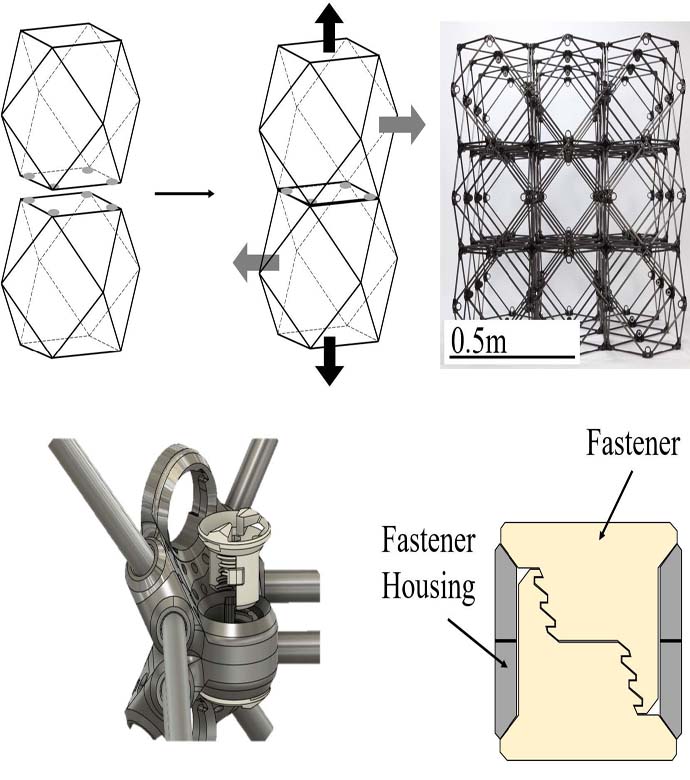
Benefits
- Enables robotic assembly: The androgynous fasteners ease of actuation (i.e., low activation force, high holding strength) and high robotic end effector engagement tolerances allow for robotic assembly by small, mobile robots
- High strength and stiffness: NASAs lightweight fasteners enable the assembly of high strength-to-mass structures for use-cases ranging from assembly of lunar habitats to terrestrial structures
- Reversibility: A reversible mechanical connection enables fastener re-use and easy reconfigurability for modular structures leveraging the fastener
- Scalability: Can be manufactured at a broad range of sizes, meaning it can be utilized for coupling modular structural components at any scale
Applications
- Autonomous robotic assembly industries
- Fastener manufacturing
- On-orbit robotic fastening of modular structural components (e.g., aerospace structures, space structures, consumer products, etc.)
- Modular reconfigurable robotics industries
Technology Details
robotics automation and control
TOP2-310
ARC-18550-1
https://ntrs.nasa.gov/citations/20200001891
Similar Results

Assemblers
Assemblers are a team of modular robots that work together to build things. Each Assembler is a stack of one or more Stewart platforms, or hexapods, made up of two plates connected by six linear actuators for movement, enabling a full six-degree-of-freedom (DOF) pose of the top plate relative to the bottom plate (see figure to the right). An end effector on each Assembler enables gripping, lifting, and welding/joining. The Assemblers system architecture features novel control algorithms and software, sensors, and communicator technology that coordinate operations of Assembler teams. The control system includes an important module for task management that estimates how many robots are needed, the optimal number of hexapods in each Assembler, and the estimated voltage needed. There are also modules for trajectory generation, joint control, sensor fusion, and fault detection. The novel control system directs the Assembler operations for high accuracy and precision, yet there is built-in dynamic resilience to failure. For example, if a single hexapod on an Assembler fails, the system deems it “rigid” in its last pose and redistributes the work to the other Assemblers.
The image below shows a storyboard of operations for how Assemblers might build a solar array. NASA has developed a hardware demo with communications between subsystems, backed up by detailed simulations of the kinematics and actuator dynamics.

Lunar Surface Manipulation System
NASA Langley developed the LSMS because of the need for a versatile system capable of performing multiple functions on the lunar surface, such as unloading components from a lander, transporting components to an operational site and installing them, and supporting service and replacement during component life. Current devices used for in-space operations are designed to work on orbit (zero g) only and thus do not have sufficient strength to operate on planetary surfaces. Traditional cranes are specialized to the task of lifting and are not capable of manipulator-type positioning operations.
The innovations incorporated into the LSMS allow it to lower payloads to the ground over a significant portion of the workspace without use of a hoist, functioning like a robot manipulator, thus providing a rigid connection and very precise control of the payload. The LSMS uses a truss architecture with pure compression and tension members to achieve a lightweight design. The innovation of using multiple spreaders (like spokes in a wheel) allows the LSMS to maintain its high structural efficiency throughout its full range of motion. Rod portions of the tension members automatically lift off and re-engage the spreaders as the joint articulates, allowing a large range of motion while maintaining mechanical advantage. In addition, the LSMS uses a quick-change device at the tip end that enables automated acquisition of end effectors or special purpose tools to increase its versatility.

Square Structural Joint with Robotic Assembly Tool
The square form joint has several novel features to improve reliability, performance and robustness. Most simply, the square tubes are stronger than round for a specified maximum cross-section dimension.
Structural benefits include nearly complete perimeter contact geometry for improved structural efficiency, improved cantilever beam response via linear bending response about y and z axis, and linear torsional response about x axis. Additionally, there is betterlinear axial response along x axis due to simple geometry and large contact surfaces, higher torsional/torque capability (about x axis), higher bending capability about all axes, higher axial capability, and is more cost effective to manufacture. It also offers a bonding strap and treated contact surfaces that provide electrical conductivity through the joint.
Switching to square cross section joints provides packaging efficiency, along with numerous improvements for robotic assembly applications such as providing rotational registration, robotically compatible tool designs, both mechanical and visual indicators to verify locking operation, preload and capture spring forces with a unique stop plate in the drive train that can be designed to default to the assembled condition without a preload, yet spring back if forced toward unlocked. After assembly, preload can be adjusted for security. Designed for robust assembly, the robotic tools are built to actuate the joint.

Multi-Link Spherical Joint
The Multi-Link Spherical Joint developed at NASA Johnson Space Center provides a substantial improvement over typical joints in which only two linearly actuated links move independently from one another. It was determined that the rotation point of a trussed link needed to be collocated at a shared point in space for maximum articulation. If not allowed separate rotation, the line of action through a universal joint and hinge acts effectively as another linkage. This leads to a much more complex and uncontrollable structure, especially when considering multiple dimensions.
Comprising the Multi-Link Spherical Joint, a spherical shell encases the cupped ends of each six possible attachments and allows each of those attachments to be independently controlled and rotated without inhibiting the motion of the others. To do this, each link is precisely limited to 15 degrees of rotation off the link centerline, thus allowing a total of 30 degrees of rotation for each link. The shell-and-cup structure can handle the loads of linear actuators that may be used to control and vary the geometry of a truss system utilizing the new joint technology. The calculated operating load that the truss system must handle can be used to scale the size of the joint, further allowing customization of any potential truss system. Additionally, the incorporated linear actuators can be controlled and powered by wiring routed through the joint without putting undo stress on the wires during operation. Accordingly, this innovative joint technology enables more efficient deployment and precise operation of articulating structures.
The Multi-Link Spherical Joint is at technology readiness level (TRL) 4 (component and/or breadboard validation in laboratory environment) and is available for patent licensing. Please note that NASA does not manufacture products itself for commercial sale.

Robonaut 2: Industrial Opportunities
NASA, GM, and Oceaneering approached the development of R2 from a dual use environment for both space and terrestrial application. NASA needed an astronaut assistant able to function in space and GM was looking for a robot that could function in an industrial setting. With this in mind, R2 was made with many capabilities that offer an enormous advantage in industrial environments. For example, the robot has the ability to retool and vary its tasks. Rather than a product moving from station to station on a conveyor with dozens of specialized robots performing unique tasks, R2 can handle several assembly steps at a single station, thereby reducing manufacturing floor space requirements and the need for multiple robots for the same activities. The robot can also be used in scenarios where dangerous chemicals, biological, or even nuclear materials are part of the manufacturing process.
R2 uses stereovision to locate human teammates or tools and a navigation system. The robot was also designed with special torsional springs and position feedback to control fine motor movements in the hands and arms. R2's hands and arms sense weight and pressure and stop when they come in contact with someone or something. These force sensing capabilities make R2 safe to work side-by-side with people on an assembly line, assisting them in ergonomically challenging tasks or working independently.
This NASA Technology is available for your company to license and develop into a commercial product. NASA does not manufacture products for commercial sale.