Search
PATENT PORTFOLIO
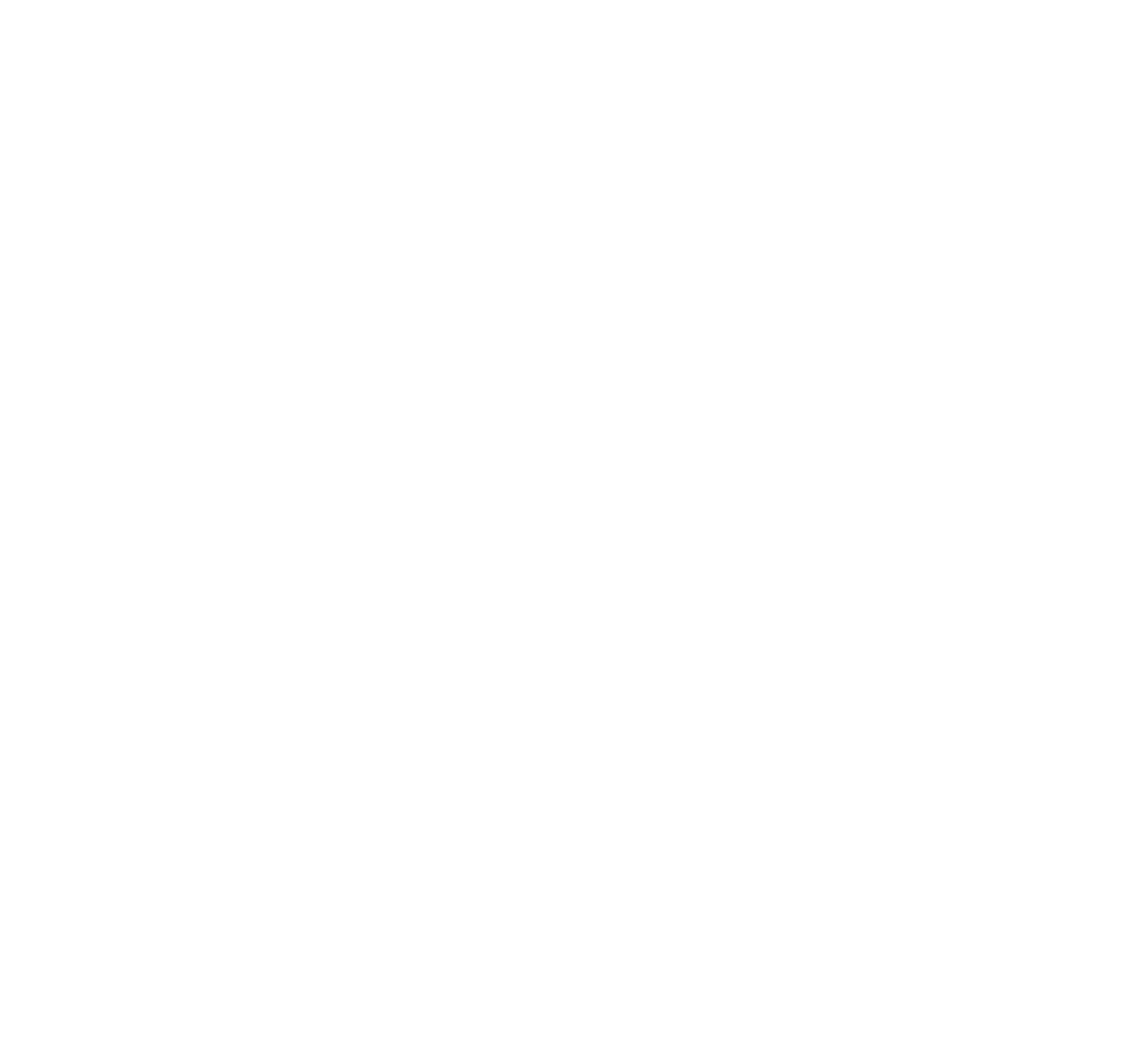
Materials and Coatings
NASA's portfolio of materials and coatings innovations represents decades of cutting-edge research and development. From high-strength metals and alloys to advanced ceramics and polymers, NASA's materials and coatings technologies offer a wide range of solutions for a variety of applications. Whether you need materials that can withstand extreme temperatures and pressures, or coatings that can protect against wear and corrosion, NASA's materials and coatings innovations have you covered.
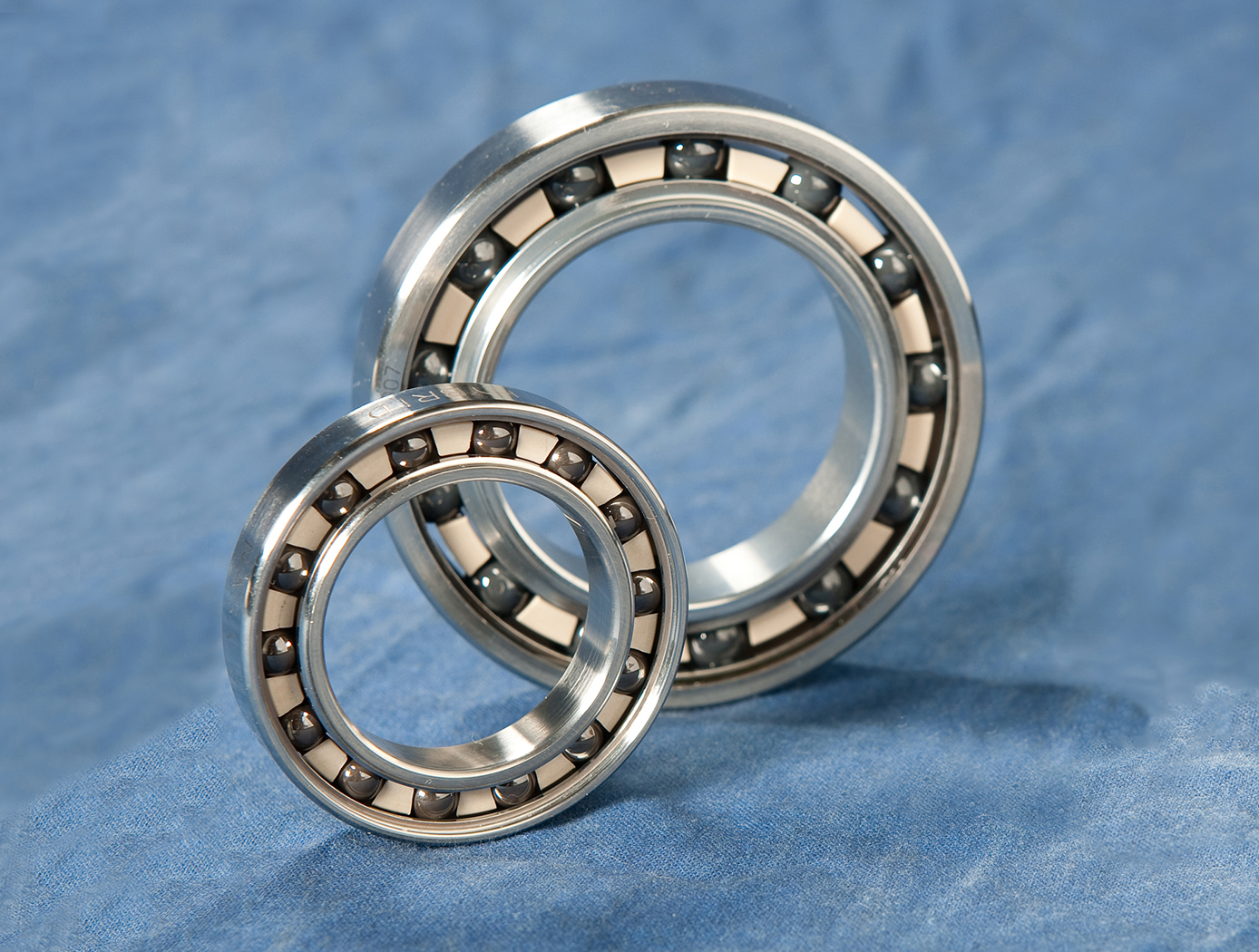
Oil-Free Lubricants
In applying PS400 using the plasma spray-coating process, a 0.010 inch layer is deposited onto a metal surface. This composite coating often includes a metallic-based binder, a metal-bonded hardener, a high-temperature lubricant, and/or a low- temperature lubricant. PS400's improved metallic-based binder alloy greatly increases the structural strength and durability of the composite with respect to the operating temperature and the bearing load, and provides superior dimensional stability. PS400's metal-bonded oxide hardening agent provides additional hardness, wear resistance, and thermal stability, while also exhibiting a low coefficient of friction when used in sliding contacts. It is also significantly less expensive in terms of both acquisition and grinding processes.
Depending on the desired environment, high- and low-temperature lubricants may be added to the composite coating. The preferred high-temperature lubricant is a metal fluoride and the optional optional low-temperature lubricant is composed of metals, such as silver or copper, that are soft enough to provide lubrication at low temperature while maintaining oxidation resistance with a sufficiently high melting point. These qualities permit the materials to be used over a broad temperature range. Once the spray coating has been applied, the metal surface is ground and polished to produce a smooth, self-lubricating surface before use. Unlike some coatings that must be diamond-ground, PS400 is readily ground with a substantially less expensive abrasive, such as silicon carbide. This grinding process generally yields a coating thickness of 200 to 400 micrometers.
In instances when a coating is not convenient or possible, powder metallurgy techniques using PM400 can be used to make freestanding self-lubricating components such as bushings and wear plates.
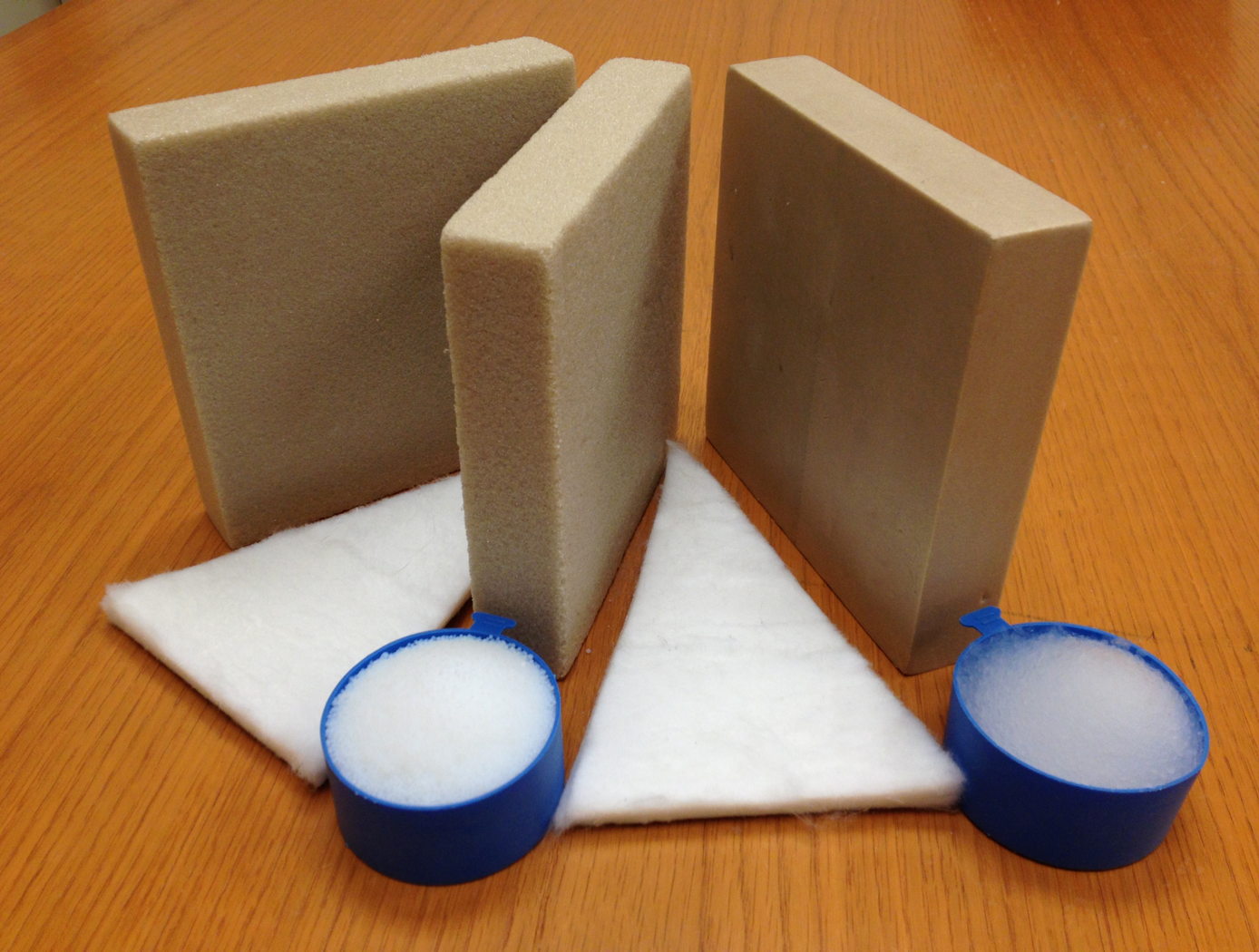
Aerofoam
The Aerofoam composites have superior thermal and acoustic insulation properties when compared to conventional polyimide foams. In addition, they provide greater structural integrity than the fragile aerogel materials can provide independently. In general, polymer foams can provide excellent thermal insulation, and polyimide foams have the additional advantage of excellent high-temperature behavior and flame resistance compared to other polymer systems (they do not burn or release noxious chemicals). Incorporating aerogel material into the polyimide foam as described by this technology creates a composite that has been demonstrated to provide additional performance gains, including 25% lower thermal conductivity with no compromise of the structural integrity and high-temperature behavior of the base polyimide foam. The structural properties of Aerofoam are variable based on its
formulation, and it can be used in numerous rigid and flexible foams of varying densities.
Aerofoam has a number of potential commercial applications, including construction, consumer appliances, transportation, electronics, healthcare, and industrial equipment. In addition, these high-performance materials may prove useful in applications that require insulation that can withstand harsh environments, including process piping, tanks for transporting and storing hot or cold fluids, ship and boat building, and aerospace applications.
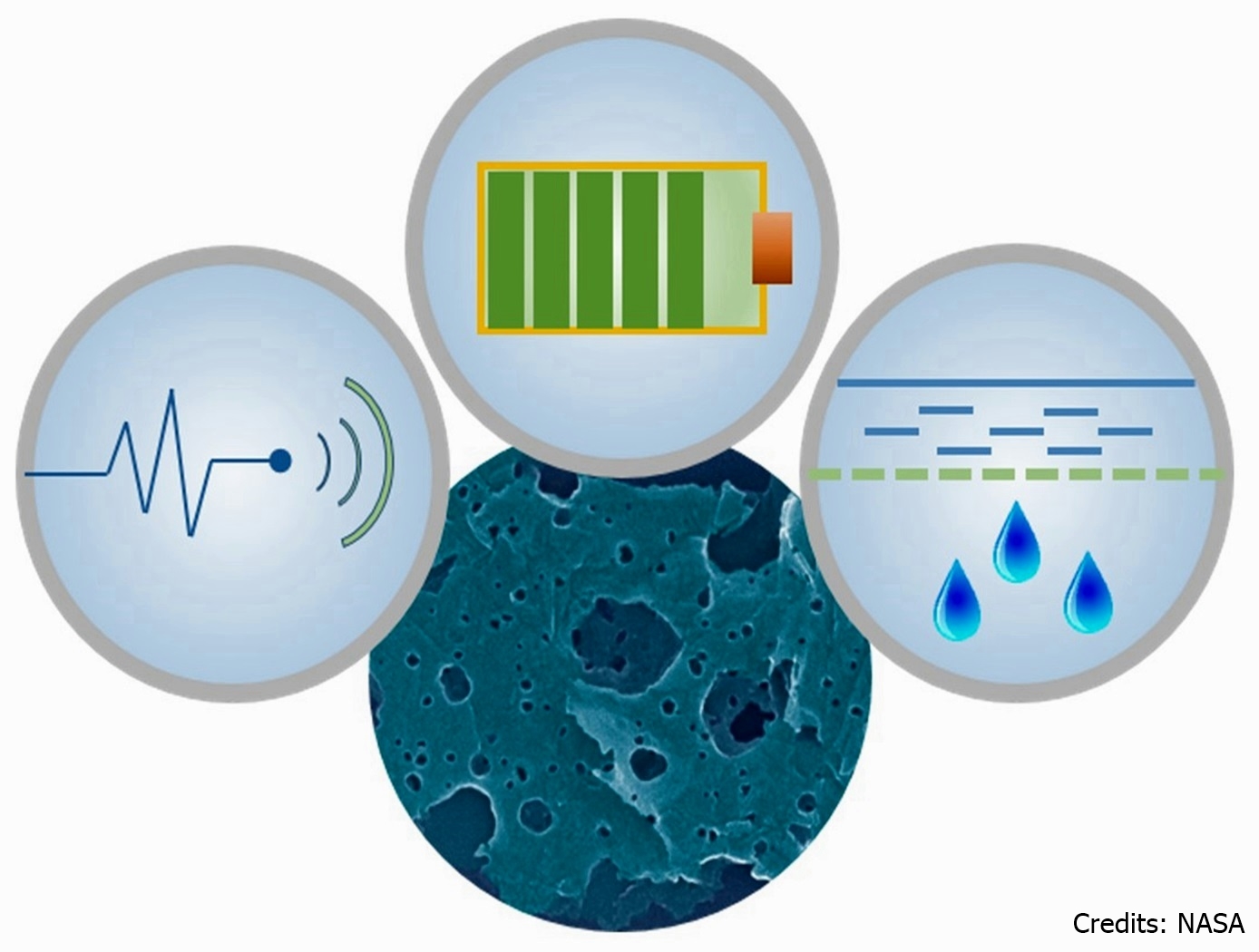
Holey Carbon Allotropes
This invention is for scalable methods that allows preparation of bulk quantities of holey nanocarbons with holes ranging from a few to over 100 nm in diameter. The first method uses metal particles as a catalyst (silver, copper, e.g.) and offers a wider range of hole diameter. The second method is free of catalysts altogether and offers more rapid processing in a single step with minimal product work-up requirements and does not require solvents, catalysts, flammable gases, additional chemical agents, or electrolysis. The process requires only commercially available materials and standard laboratory equipment; and, it is scalable. Properties that can be controlled include: surface area, pore volume, mechanical properties, electrical conductivity, and thermal conductivity.

Fine-Grained Targets
The fine-grained targets are a matrix of a prismatic edge natural flake graphite that produces strong structural pi bonds when pressed at room temperature. The targets have an approximate grain size of 2 microns. The graphite provides a carbon source that is combined with a metal catalyst made of powder forge nickel and powder forge cobalt. Previously used targets involved pressing and binding targets with Dylon carbon cement. These particles had an approximate 200-micron particle size, which led to large regions of uncatalyzed target. The new metal powders are small (~0.5 microns), highly dispersible, and two orders of magnitude less expensive. The powders are mixed in appropriate quantities and subjected to low-energy ball-milling to
ensure mingling. Targets undergo additional preparatory steps, including high pressure processing. The targets are then subjected to a series of ultrafast infrared laser light pulses at a high repetition rate to vaporize the layers of the spinning targets and create a plume of nanotubes.
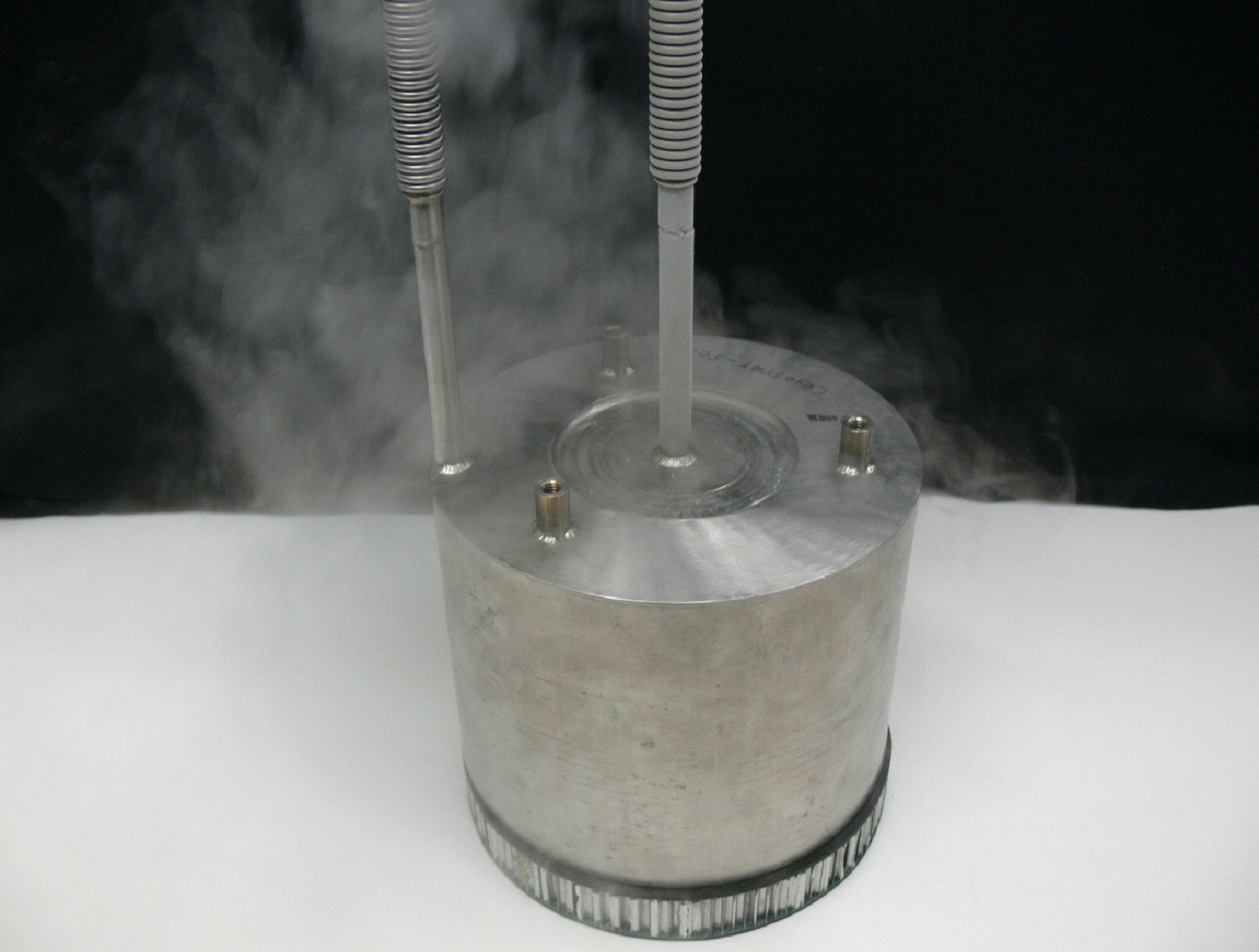
Adaptive Thermal Management System
Efficient thermal management has long been an issue in both commercial systems and in the extreme environments of space. In space exploration and habitation, significant challenges are experienced in providing fluid support systems such as cryogenic storage, life support, and habitats; or thermal control systems for launch vehicle protection, environmental heat management, or electronic instruments. Furthermore, these systems operate in dynamic, transient modes and often under extremes of temperature or pressure. The current technical requirements associated with the thermal management of these systems result in control issues as well as significant life-cycle costs.
To combat these issues, the Adaptive Thermal Management System (ATMS) was developed to help provide the capability for tanks, structural walls, or composite substrate materials to switch functionality (conductive or insulative) depending on environmental conditions or applied stimuli. As a result, the ATMS provides the ability to adapt between both heating and cooling modes within a single system. For example, shape memory alloy (SMA) elements are used to actuate at certain design temperatures to create a conductive bridge between two metal plates allowing broad-area heat rejection from the hotter surface. Upon cooling to the lower design set-point, the SMA elements return to their original shapes, thereby breaking the conductive path and returning the system to its overall insulative state.
This technology has the potential to be applied to any system that would have the need for a self-regulating thermal management system that allows for heat transfer from one side to another.
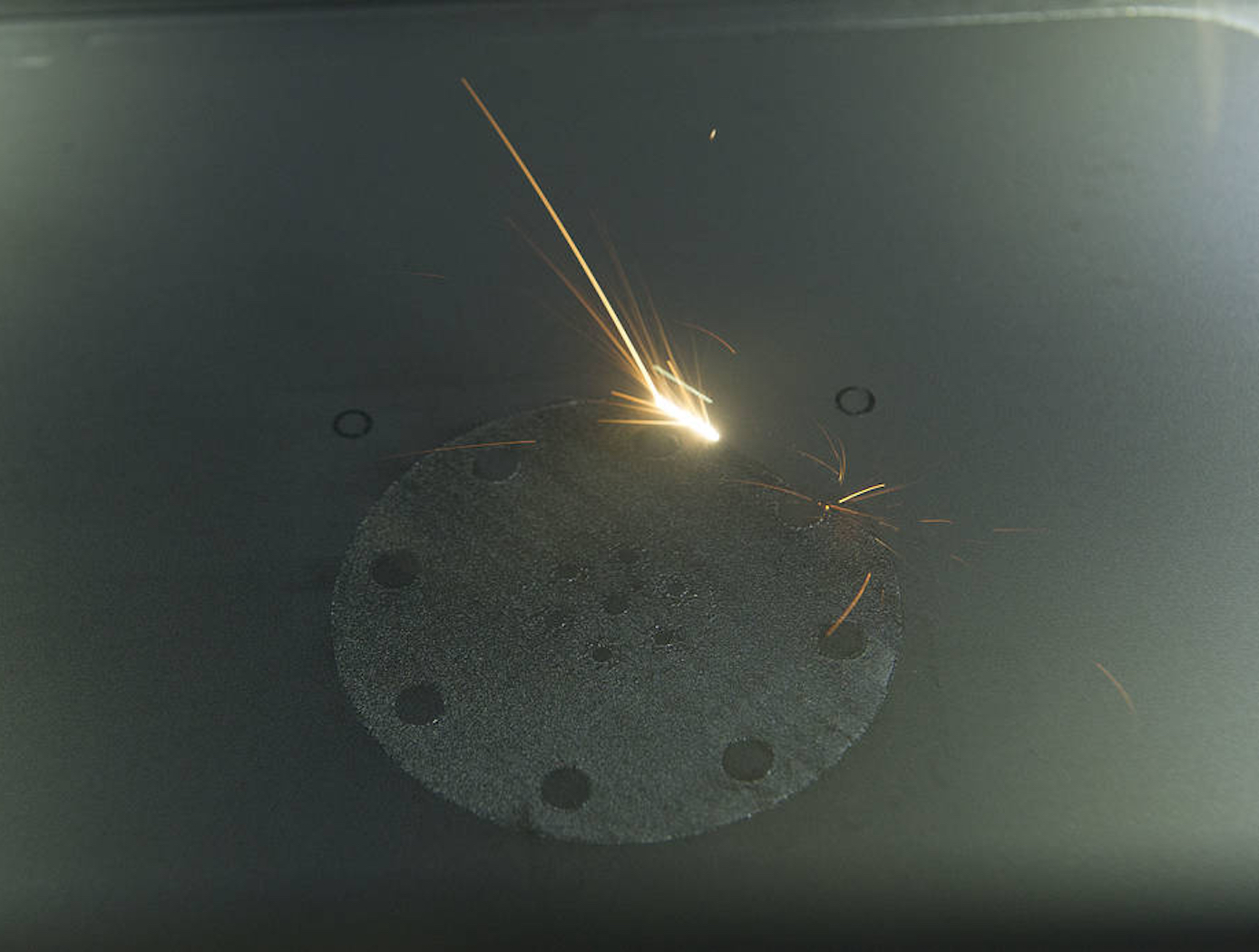
3D-Printed Composites for High Temperature Uses
NASA's technology is the first successful 3D-printing of high temperature carbon fiber filled thermoset polyimide composites. Selective Laser Sintering (SLS) of carbon-filled RTM370 is followed by post-curing to achieve higher temperature capability, resulting in a composite part with a glass transition temperature of 370 °C.
SLS typically uses thermoplastic polymeric powders and the resultant parts have a useful temperature range of 150-185 °C, while often being weaker compared to traditionally processed materials. Recently, higher temperature thermoplastics have been manufactured into 3D parts by high temperature SLS that requires a melting temperature of 380 °C, but the usable temperature range for these parts is still under 200 °C.
NASA's thermoset polyimide composites are melt-processable between 150-240 °C, allowing the use of regular SLS machines. The resultant parts are subsequently post-cured using multi-step cycles that slowly heat the material to slightly below its glass transition temperature, while avoiding dimensional change during the process. This invention will greatly benefit aerospace companies in the production of parts with complex geometry for engine components requiring over 300 °C applications, while having a wealth of other potential applications including, but not limited to, printing legacy parts for military aircraft and producing components for high performance electric cars.

New Resin Systems for Thermal Protection Materials
This method produces a low density ablator similar to Phenolic Impregnated Carbon Ablator (PICA) using a cyanate ester and phthalonitrile resin system, rather than the heritage phenolic resin. Cyanate ester resin systems can be cured in a carbon matrix and generate high surface area structure within the carbon fibers. This helps to reduce the thermal conductivity of the material which is one of the key requirements of thermal protection system (TPS) materials. The material has densities ranging from 0.2 to .35 grams per cubic centimeter. NASA has successfully processed the cyanate ester and phthalonitrile resins with a morphology similar to that of the phenolic phase in PICA, but with more advanced properties such as high char stability, high char yield, and high thermal stability. This new generation of TPS materials has the same microstructure as heritage PICA, but improved characteristics of PICA such as increased char yield, increased char stability, increased thermal stability and increased glass transition temperature.
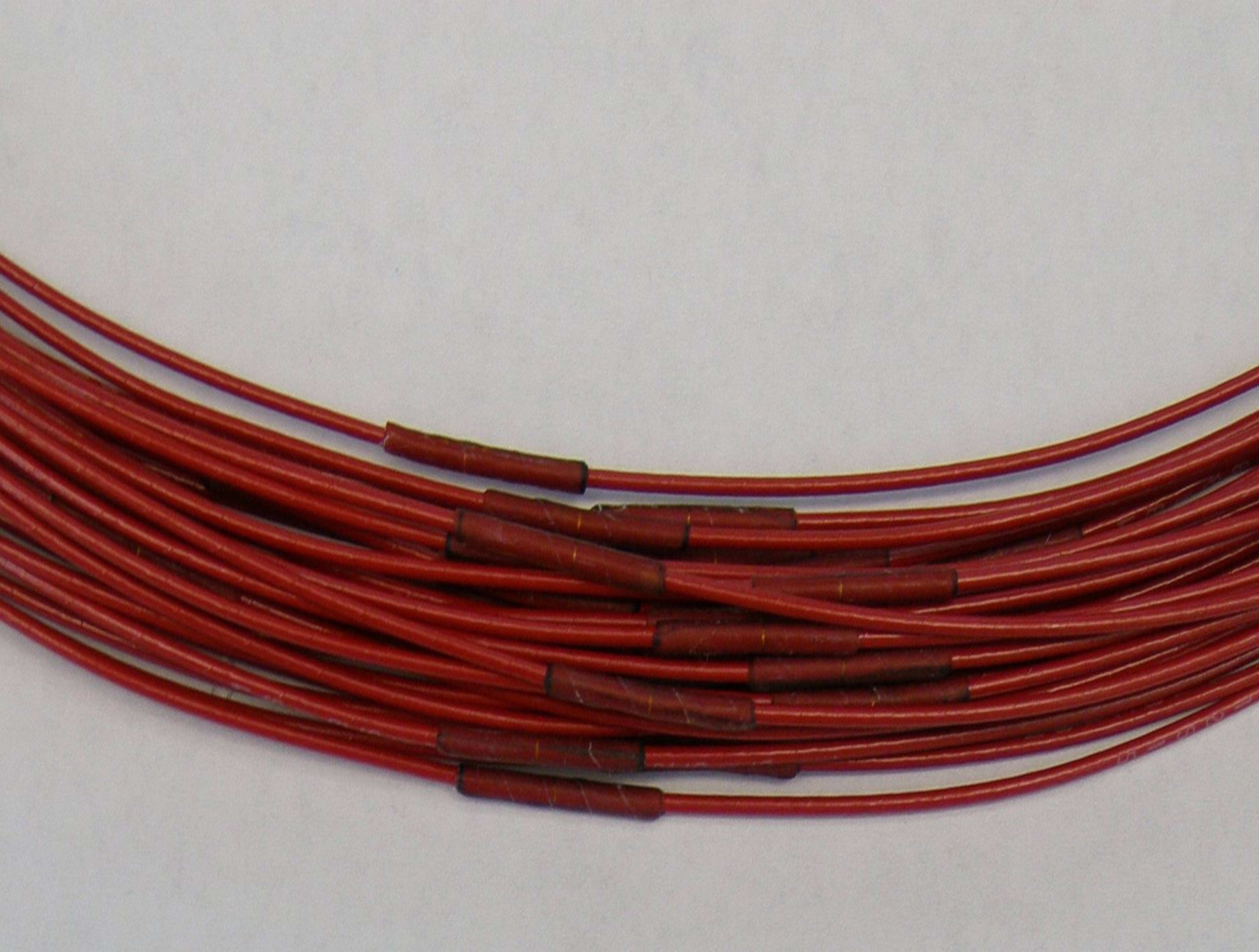
Polyimide Wire Insulation Repair System
A major limitation of current aerospace wire insulation is that it tends to crack and fray as it ages and is easily damaged. Generally, it is more cost-effective to repair wire insulation than to replace a section of the wire (or bundle) itself. Current repair methods include a tape wrap repair and a heat shrink repair. These methods have a number of drawbacks: susceptibility to vibration, fluid intrusion, and other mechanical stresses. The repair patch/material can loosen or separate, exposing the bare metal conductor or opening the polyimide insulation to more damage at the interface.
The technology developed by KSC is a flexible polyimide film patch (either wrap or sleeve) that is heated with a custom heating tool to melt, flow, and cure the film. The new technology results in hermetically sealed, permanent repairs that are much more flexible and less intrusive than repairs made using current practices. The repair remains flexible after application, has no limit in length or bend radius, and retains the high-temperature exposure of the original polyimide insulation. Extensive testing by NASA and NAVAIR has demonstrated that these repairs comply with industry standards for tensile strength, electrical resistivity, voltage breakdown, solvent resistance, and flammability. This system is adaptable and may also be used on larger-gauge wiring, as well as flat-ribbon wire harnesses and twisted shielded wires.
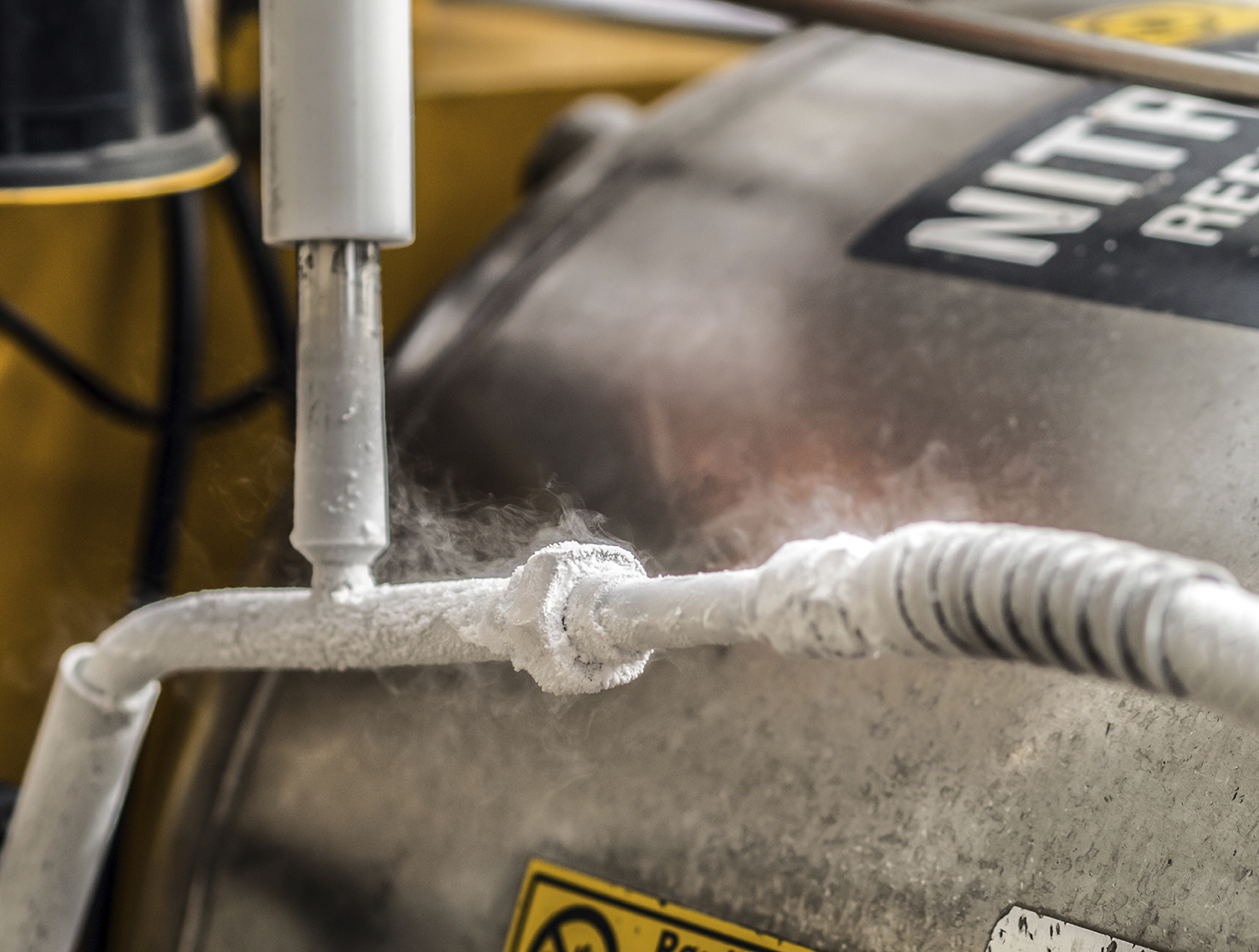
Polyamide Aerogels
Polyamides are polymers that are similar to polyimides (another polymer that has been developed for use in aerogels). However, because the amide link is a single chain while the imide link is a ring structure, polyamide aerogels can be made less stiff than polyimides, even though a similar fabrication process is used. The precursor materials can be made from any combination of diamine and diacid chloride. Furthermore, NASA Glenn researchers have found methods for using combinations of diamines and disecondary amines to produce polyamide aerogels with tunable glass transition temperatures, for greater control of features such as flexibility or water-resistance.
In the first step of the fabrication process, an oligomeric solution is produced that is stable and can be prepared and stored indefinitely as stock solutions prior to cross-linking. This unique feature allows for the preparation and transport of tailor-made polyamide solutions, which can later be turned into gels via the addition of a small amount of cross-linker. When the cross-linking agent is added, the solution can be cast in a variety of forms such as thin films and monoliths. To remove the solvent, one or more solvent exchanges can be performed, and then the gel is subjected to supercritical drying to form a polyamide aerogel. NASA Glenn's polyamide aerogels can be fully integrated with the fabrication techniques and products of polyimide aerogel fabrication, so hybrid materials which have the properties of both classes are easily prepared. As the first aerogels to be composed of cross-linked polyamides, these materials combine flexibility and transparency in a way that sets them apart from all other polymeric aerogels.
View more patents