Search
PATENT PORTFOLIO
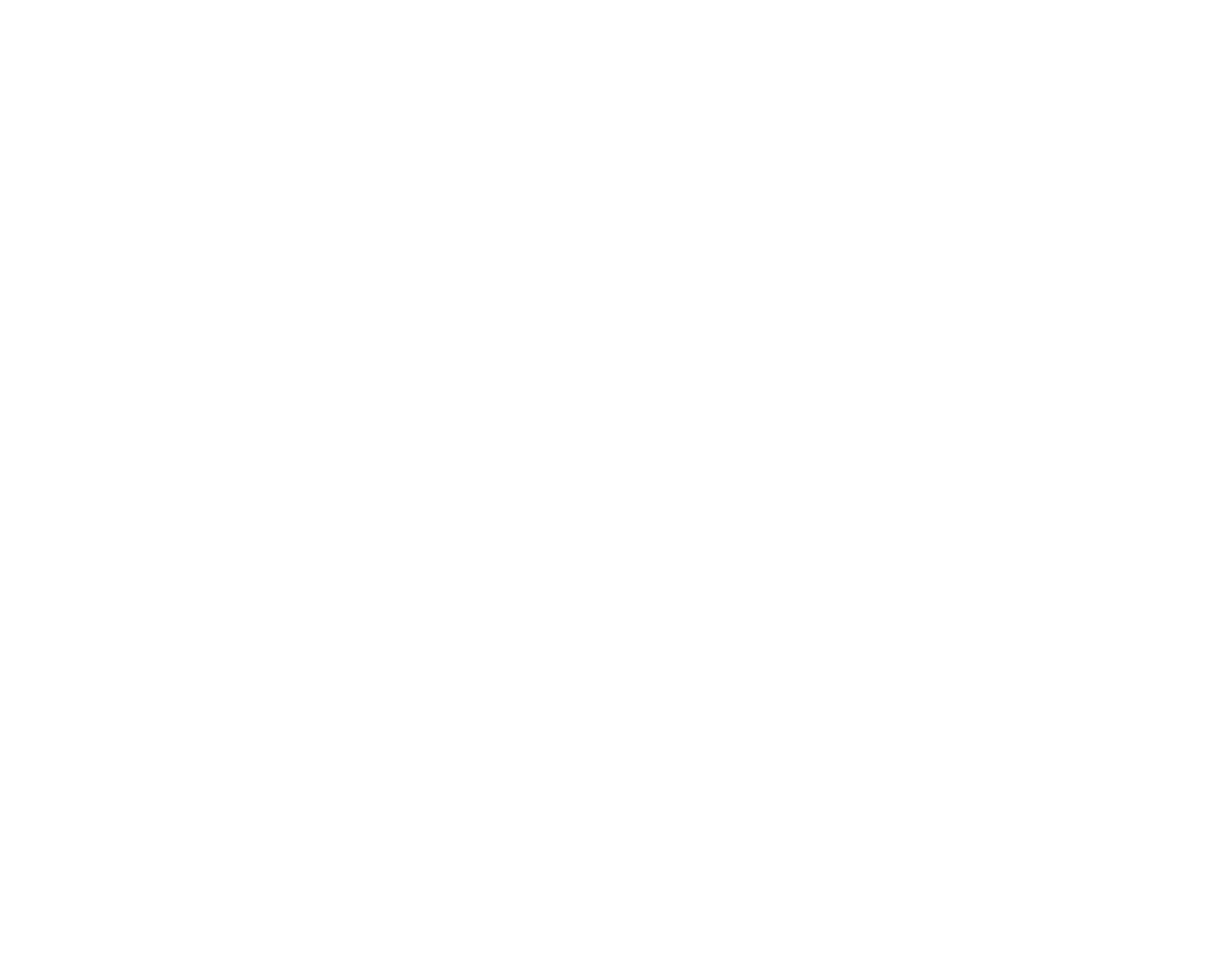
Optics
If you're looking for the most advanced optics technology available, look no further than NASA's extensive portfolio. From high-precision imaging systems to state-of-the-art lasers and optical components, NASA's optics technologies can help revolutionize a wide range of industries and drive the next generation of innovation.
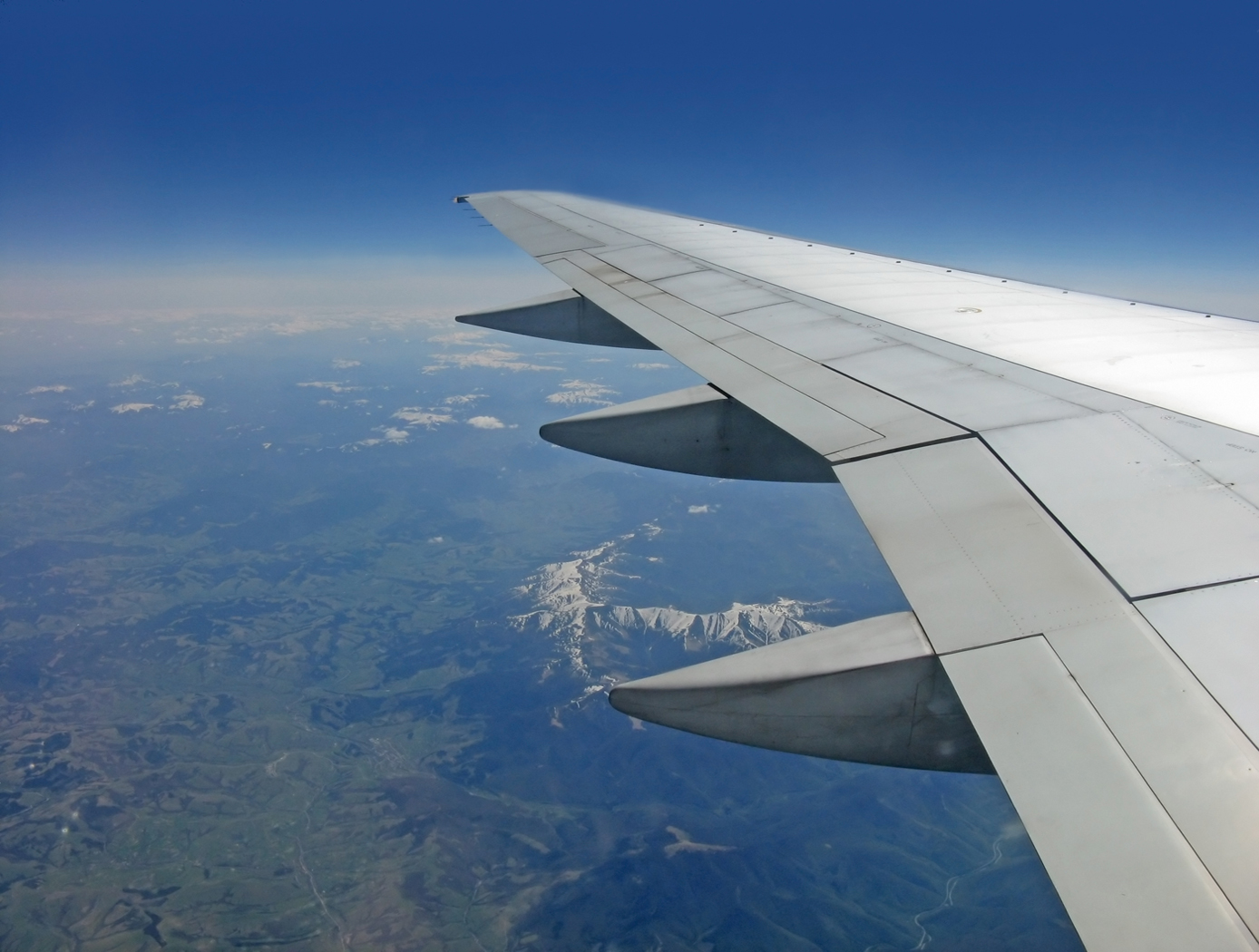
Strobing to Mitigate Vibration for Display Legibility
The dominant frequency of the vibration that requires mitigation can be known in advance, measured in real time, or predicted with simulation algorithms. That frequency (or a lower frequency multiplier) is then used to drive the strobing rate of the illumination source. For example, if the vibration frequency is 20 Hz, one could employ a strobe rate of 1, 2, 4, 5, 10, or 20 Hz, depending on which rate the operator finds the least intrusive. The strobed illumination source can be internal or external to the display.
Perceptual psychologists have long understood that strobed illumination can freeze moving objects in the visual field. This effect can be used for artistic effect or for technical applications. The present innovation is instead applicable for environments in which the human observer rather than just the viewed object undergoes vibration. Such environments include space, air, land, and sea vehicles, or on foot (e.g., walking or running on the ground or treadmills). The technology itself can be integrated into handheld and fixed display panels, head-mounted displays, and cabin illumination for viewing printed materials.
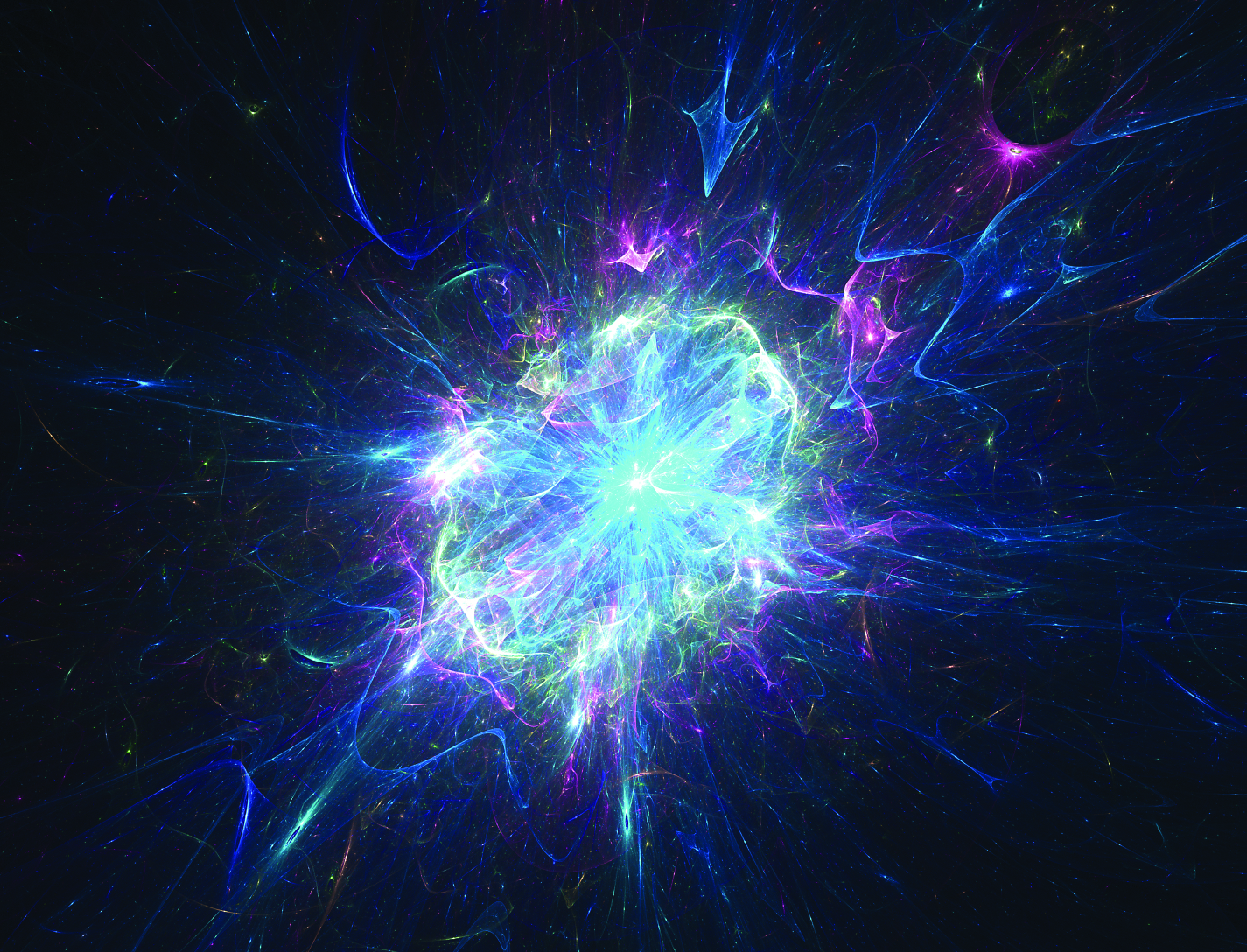
Nested Focusing Optics for Compact Neutron Sources
Conventional neutron beam experiments demand high fluxes that can only be obtained at research facilities equipped with a reactor source and neutron optics. However, access to these facilities is limited. The NASA technology uses grazing incidence reflective optics to produce focused beams of neutrons (Figure 1) from compact commercially available sources, resulting in higher flux concentrations. Neutrons are doubly reflected off of a parabolic and hyperbolic mirror at a sufficiently small angle, creating neutron beams that are convergent, divergent, or parallel. Neutron flux can be increased by concentrically nesting mirrors with the same focal length and curvature, resulting in a convergence of multiple neutron beams at a single focal point. The improved flux from the compact source may be used for non-destructive testing, imaging, and materials analysis.
The grazing incidence neutron optic mirrors are fabricated using an electroformed nickel replication technique developed by NASA and the Harvard-Smithsonian Center for Astrophysics (Figure 2). A machined aluminum mandrel is super-polished to a surface roughness of 3-4 angstroms root mean square and plated with layers of highly reflective nickel-cobalt alloy. Residual stresses that can cause mirror warping are eliminated by periodically reversing the anode and cathode polarity of the electroplating system, resulting in a deformation-free surface. The fabrication process has been used to produce 0.5 meter and 1.0 meter lenses.
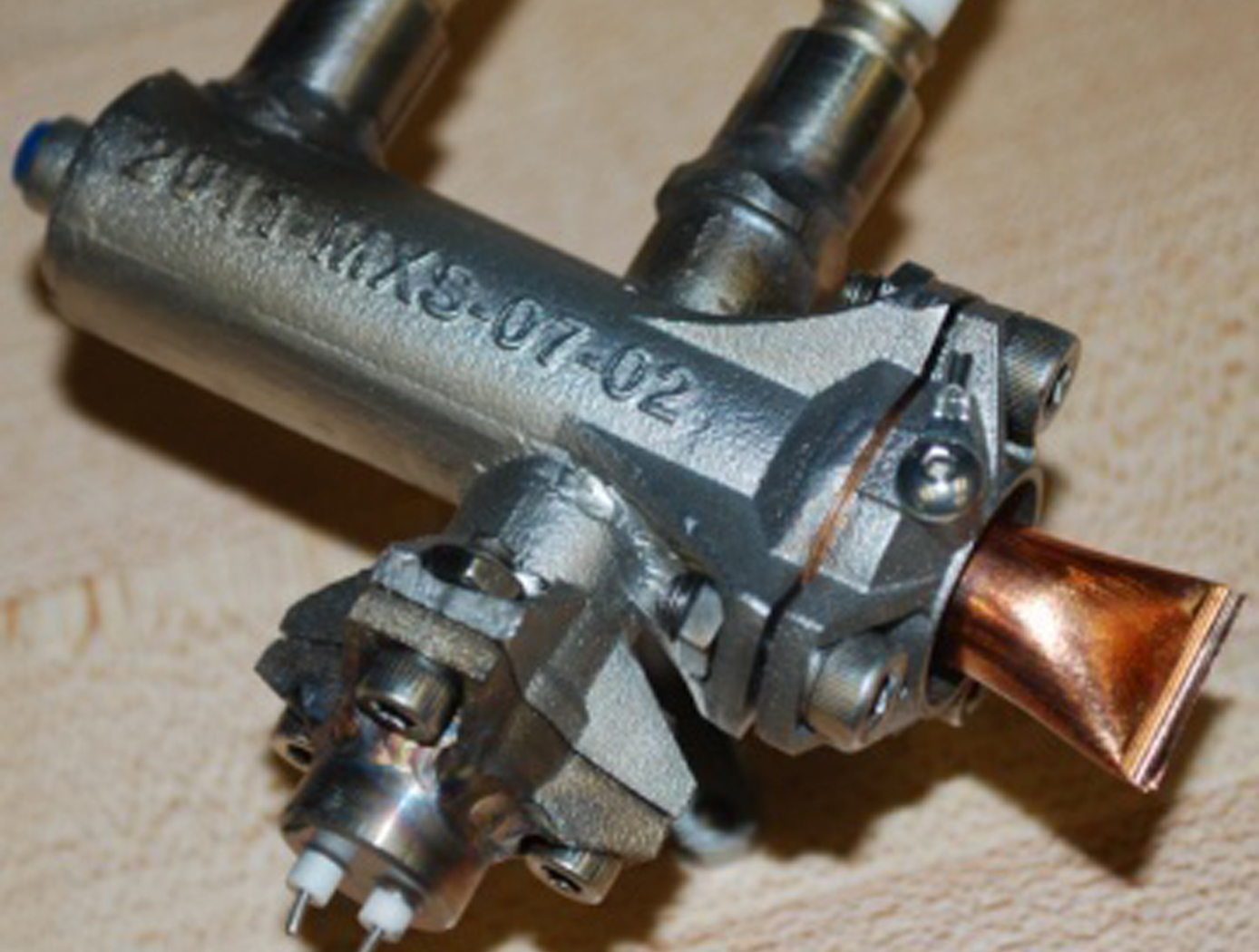
Miniaturized High-Speed Modulated X-Ray Source (MXS)
The MXS produces electrons by shining UV light from an LED onto a photocathode material such as magnesium. The electrons are then accelerated across several kV and into a chosen target material; deceleration produces X-rays characteristic of the target. The MXS uses an electron multiplier for high X-ray production efficiency.
The MXS is more compact, rugged, and power-efficient than standard X-ray sources. It can be manufactured using commercially available components and 3D printed housing, resulting in a low cost to manufacture. Unlike traditional X-ray sources, the MXS does not require a filament or vacuum and cooling systems. Most importantly, enabling rapid and arbitrary modulation allows using X-rays in the time domain, a new dimension to X-ray applications.
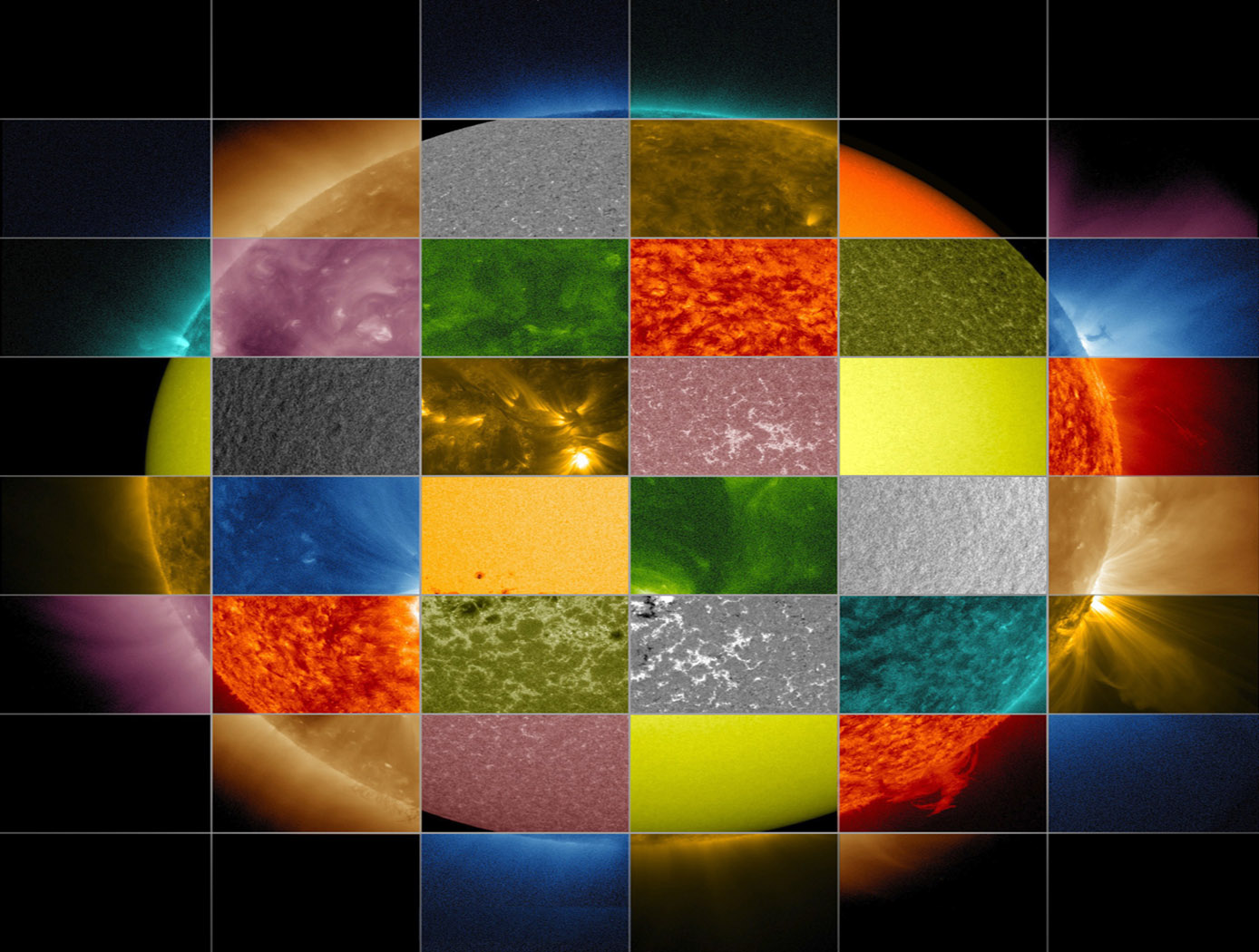
Self-Phase-Locked Distributed Gain Laser Architecture
NASA Goddard Space Flight Center has developed a laser architecture to coherently combine energy from spatially distributed gain sources. Using a combination of lenslet arrays (to split and combine separate beams) or diffractive optical elements, each source can be phase-matched into an effective single source.
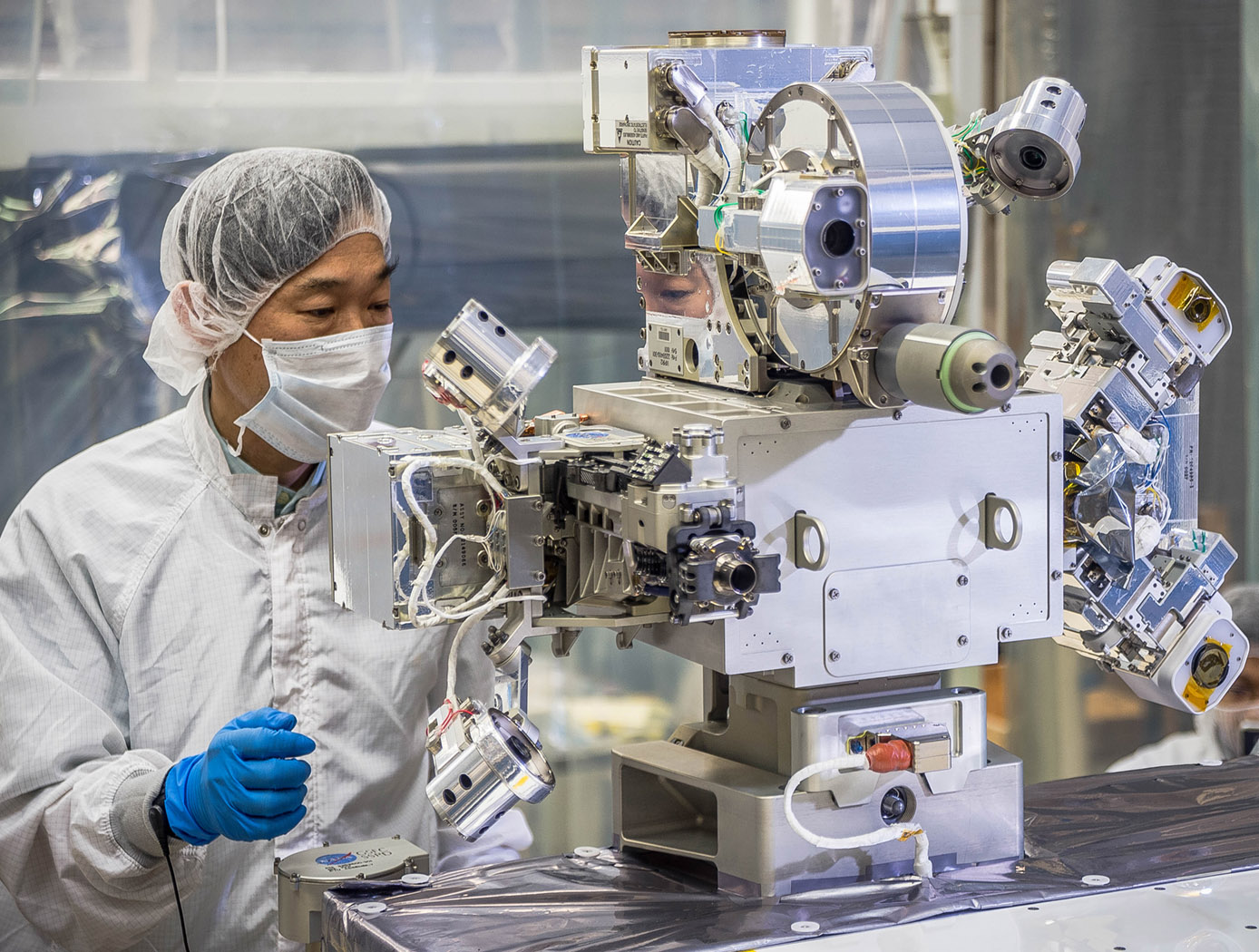
Goddard's Reconfigurable Laser Ranger (GRLR)
NASA Goddard Space Flight Center has developed a low cost, modular, and flexible space flight laser range finder consisting of optics, electronics, and interfaces for satellite servicing missions (i.e. Restore-L) using customized optics. Built upon previous NASA technologies, the system also consists of a high dynamic range receiver and adjustable laser for a wide range of measurements (i.e. multiples of km to sub-meter).

Video Acuity Measurement System
The Video Acuity metric is designed to provide a unique and meaningful measurement of the quality of a video system. The automated system for measuring video acuity is based on a model of human letter recognition. The Video Acuity measurement system is comprised of a camera and associated optics and sensor, processing elements including digital compression, transmission over an electronic network, and an electronic display for viewing of the display by a human viewer. The quality of a video system impacts the ability of the human viewer to perform public safety tasks, such as reading of automobile license plates, recognition of faces, and recognition of handheld weapons. The Video Acuity metric can accurately measure the effects of sampling, blur, noise, quantization, compression, geometric distortion, and other effects. This is because it does not rely on any particular theoretical model of imaging, but simply measures the performance in a task that incorporates essential aspects of human use of video, notably recognition of patterns and objects. Because the metric is structurally identical to human visual acuity, the numbers that it yields have immediate and concrete meaning. Furthermore, they can be related to the human visual acuity needed to do the task. The Video Acuity measurement system uses different sets of optotypes and uses automated letter recognition to simulate the human observer.

On-demand, Dynamic Reconfigurable Broadcast Technology for Space Laser Communication
NASA Goddard Space Flight Center has developed a configurable phase mirror system that can address likely obstacles in space optical communications. Through using miniature adjustable mirrors and programmed phase delays to diffract a single communication beam, numerous diffracted beams can be sent to other satellites in various directions for communication and tracking. The initial laser beams wave profile can be dynamically regulated through a fast Fourier transform (FFT) so that when it reaches its desired destination, it forms an intended illuminated spot at the target satellite. Since all the diffracted beams share the same phase mirror, the antenna gain needed to broadcast these beams does not require a multiplied aperture.
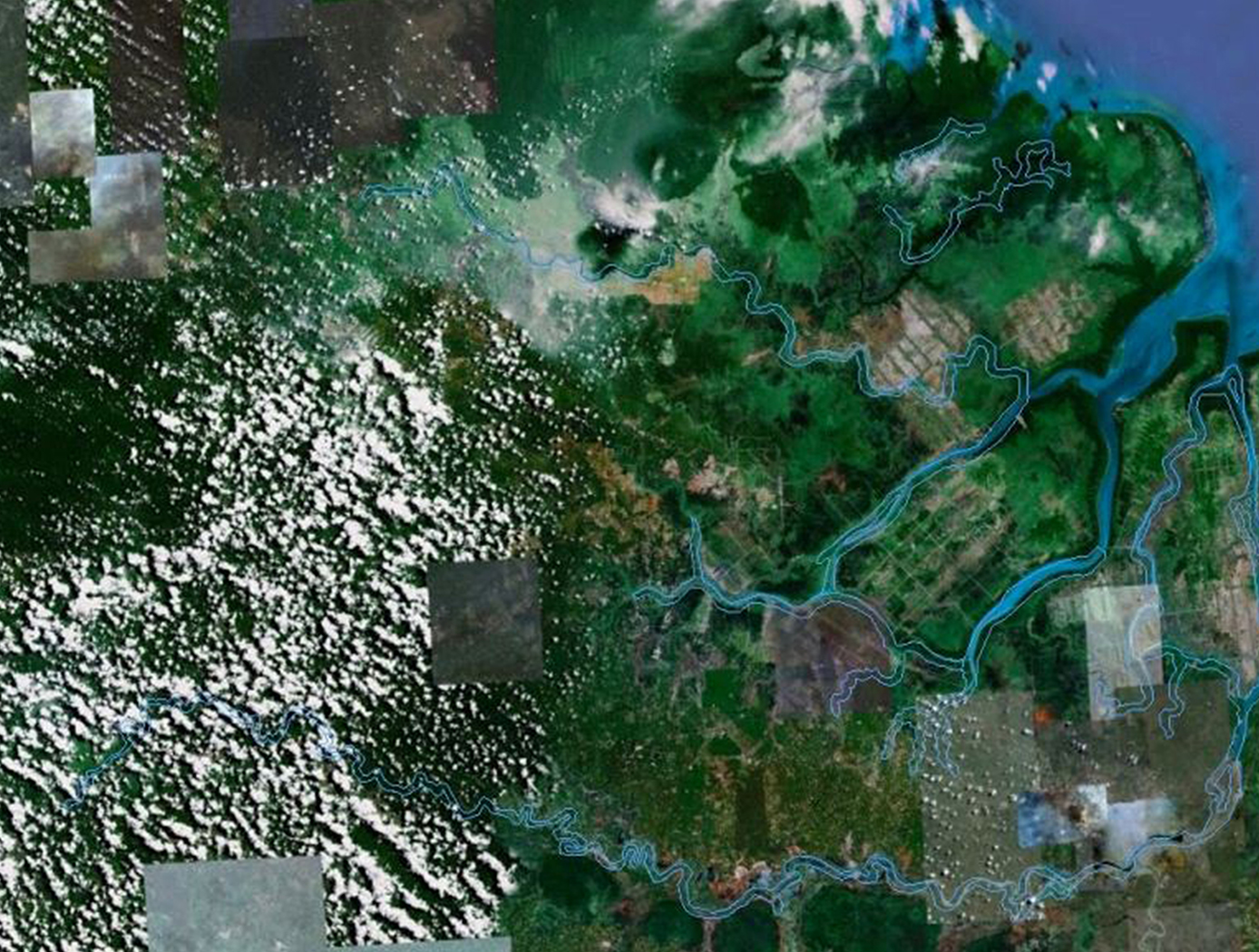
Digital Beamforming Interferometry
NASA Goddard Space Flight Center (GSFC) has developed a new approach that uses a single phased array antenna and a single pass configuration to generate interferograms, known as Digital Beamforming Interferometry. A digital beamforming radar system allows the implementation of non-conventional radar techniques, known as Digital Beamforming Synthetic Aperture Radar Multi-mode Operation (DBSAR).
DBSAR is an L-Band airborne radar that combines advanced radar technology with the ability to implement multimode remote sensing techniques, including several variations of SAR, scatterometry over multiple beams, and an altimeter mode. The Multiple channel data acquired with a digital beamformer systems allows the synthesis of beams over separate areas of the antenna, effectively dividing the single antenna into two antennas. The InSAR technique is then achieved by generating interferograms from images collected with each of the antennas. Since the technique is performed on the data, it allows for synthesizing beams in different directions (or look angles) and performs interferometry over large areas.
Digital Beamforming Interferometry has potential in many areas of radar applications. For example, NASA GSFC innovators developed the first P-Band Digital Beamforming Polarimetric Interferometric SAR Instrument to measure ecosystem structure, biomass, and surface water.

Non-Scanning 3D Imager
NASA Goddard Space Flight Center's has developed a non-scanning, 3D imaging laser system that uses a simple lens system to simultaneously generate a one-dimensional or two-dimensional array of optical (light) spots to illuminate an object, surface or image to generate a topographic profile.
The system includes a microlens array configured in combination with a spherical lens to generate a uniform array for a two dimensional detector, an optical receiver, and a pulsed laser as the transmitter light source. The pulsed laser travels to and from the light source and the object. A fraction of the light is imaged using the optical detector, and a threshold detector is used to determine the time of day when the pulse arrived at the detector (using picosecond to nanosecond precision). Distance information can be determined for each pixel in the array, which can then be displayed to form a three-dimensional image.
Real-time three-dimensional images are produced with the system at television frame rates (30 frames per second) or higher.
Alternate embodiments of this innovation include the use of a light emitting diode in place of a pulsed laser, and/or a macrolens array in place of a microlens.
View more patents