Nested Focusing Optics for Compact Neutron Sources
optics
Nested Focusing Optics for Compact Neutron Sources (MFS-TOPS-40)
Enabling high performance neutron imaging and analysis
Overview
NASA's Marshall Space Flight Center has developed novel neutron grazing incidence optics for use with small-scale portable neutron generators. The technology was developed to enable the use of commercially available neutron generators for applications requiring high flux densities, including high performance imaging and analysis. Nested grazing incidence mirror optics, with high collection efficiency, are used to produce divergent, parallel, or convergent neutron beams. Ray tracing simulations of the system (with source-object separation of 10m for 5 meV neutrons) show nearly an order of magnitude neutron flux increase on a 1-mm diameter object. The technology is a result of joint development efforts between NASA and MIT researchers seeking to maximize neutron flux from diffuse sources for imaging and testing applications.
The Technology
Conventional neutron beam experiments demand high fluxes that can only be obtained at research facilities equipped with a reactor source and neutron optics. However, access to these facilities is limited. The NASA technology uses grazing incidence reflective optics to produce focused beams of neutrons (Figure 1) from compact commercially available sources, resulting in higher flux concentrations. Neutrons are doubly reflected off of a parabolic and hyperbolic mirror at a sufficiently small angle, creating neutron beams that are convergent, divergent, or parallel. Neutron flux can be increased by concentrically nesting mirrors with the same focal length and curvature, resulting in a convergence of multiple neutron beams at a single focal point. The improved flux from the compact source may be used for non-destructive testing, imaging, and materials analysis.
The grazing incidence neutron optic mirrors are fabricated using an electroformed nickel replication technique developed by NASA and the Harvard-Smithsonian Center for Astrophysics (Figure 2). A machined aluminum mandrel is super-polished to a surface roughness of 3-4 angstroms root mean square and plated with layers of highly reflective nickel-cobalt alloy. Residual stresses that can cause mirror warping are eliminated by periodically reversing the anode and cathode polarity of the electroplating system, resulting in a deformation-free surface. The fabrication process has been used to produce 0.5 meter and 1.0 meter lenses.
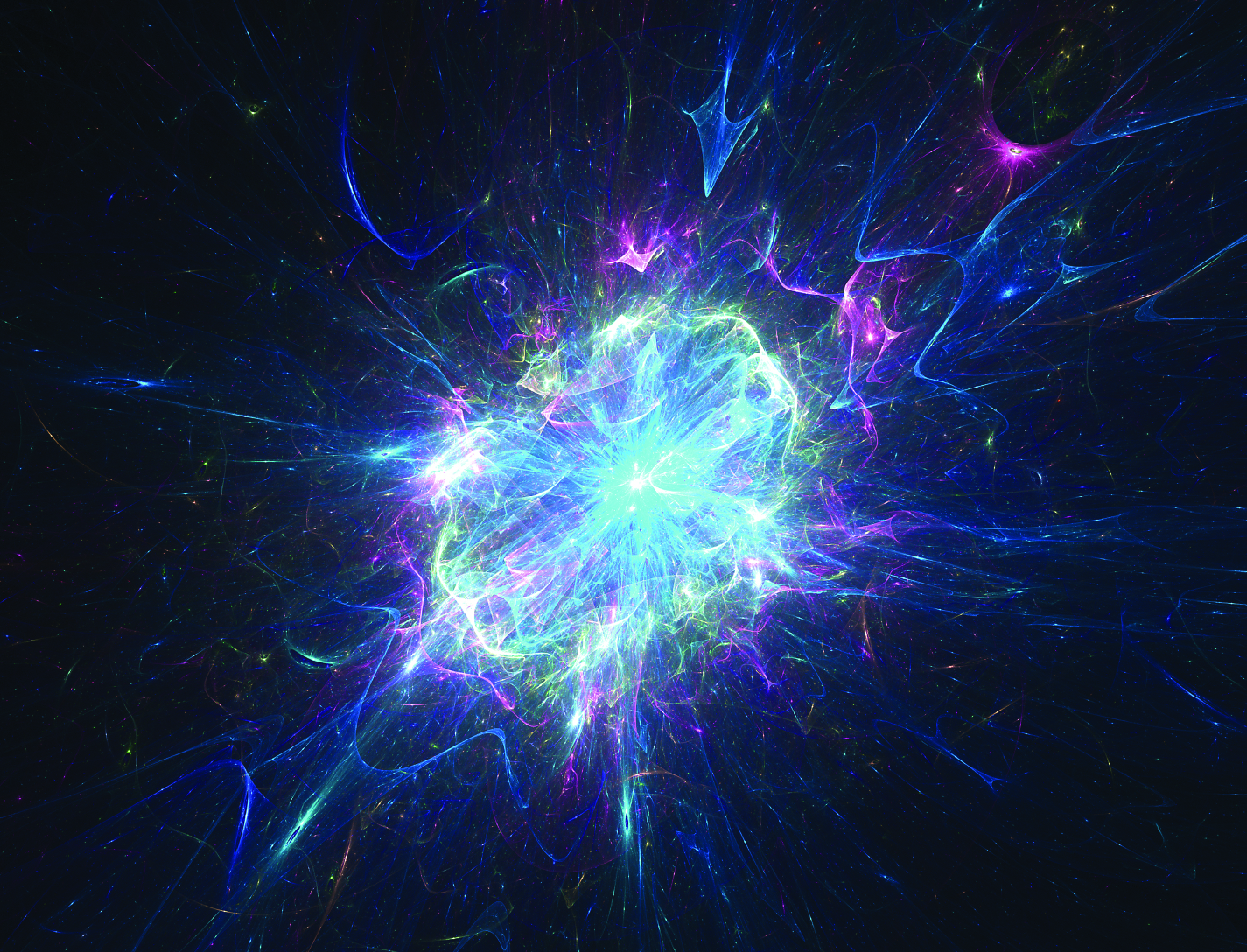
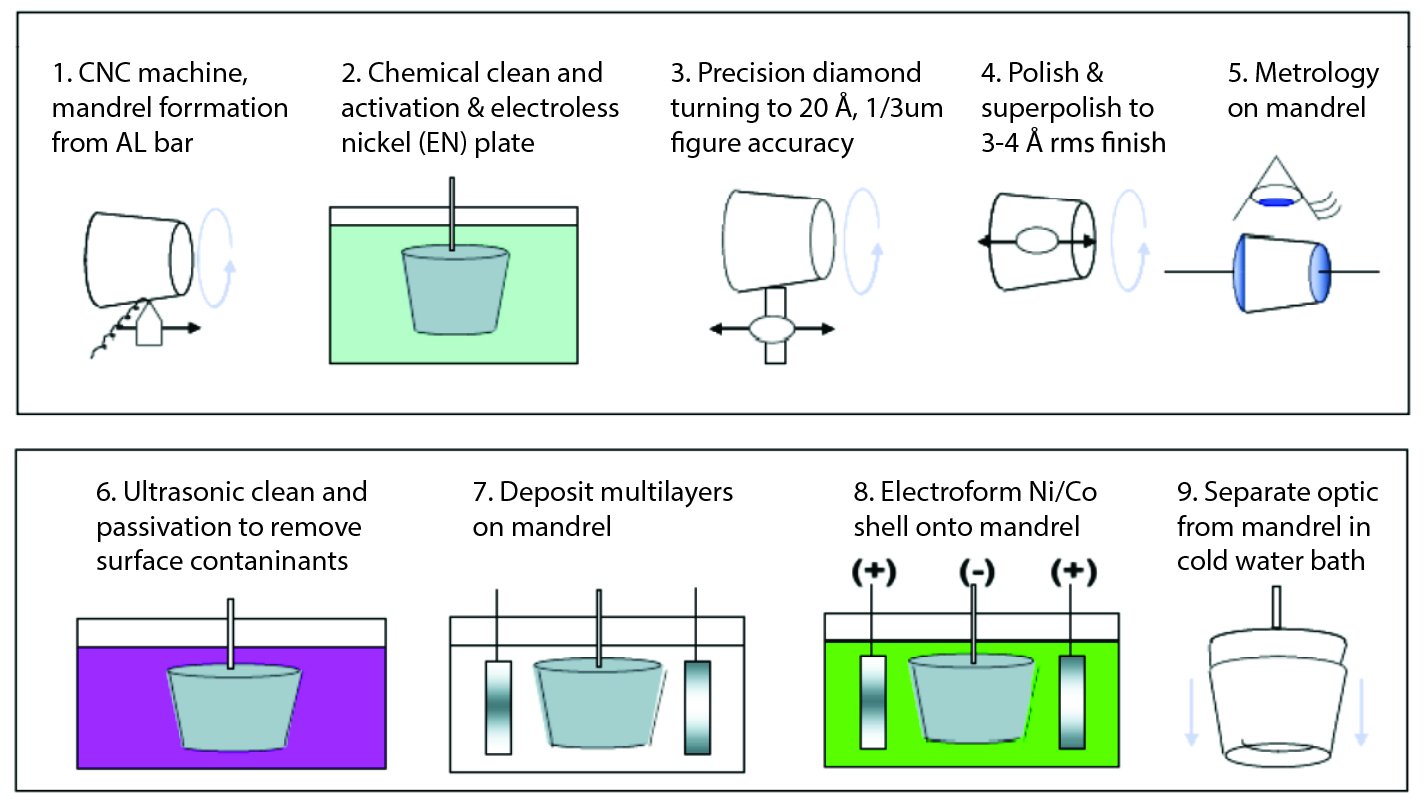
Benefits
- Improved performance: enabling use of smaller neutron sources for imaging and analytical techniques
- Increased flexibility: removable mirrors enables easy experimental flux adjustments
- Reduced optic cost: novel replication technique enables copies from a single master
- Increased collection efficiency: superior to neutron focusing guides and Kirkpatrick-Baez mirrors
Applications
- Non-destructive inspection for jet engine turbine blades, fuel cells, archaeological artifacts, and weld inspections
- Analytical techniques for small angle neutron scattering (SANS), time-offlight spectroscopy, convergent beam crystallography, and inelastic scattering instruments
Tags:
|
Similar Results

Improved Efficiency in Nuclear Propulsion
Current nuclear propulsion technologies do not utilize the additional energy expelled as gamma ray radiation during nuclear fission. This results in the loss of 6.5% of the total fission energy, which could be used to improve propulsion capabilities. The NTAC Augmented Nuclear Electric Propulsion and/or Nuclear Thermal Propulsion design is more efficient than existing technology and captures an additional 6.5% (13.3 MeV) of radiation energy that is currently lost to radiation shielding. In this design the NTAC cell structure surrounds the TRISO (TRi-structural ISOtropic particle fuel) bead-filled rocket chamber, covering all sides and the top of the chamber, therefore capturing additional energy. This structure allows one-way energy flow to then be expelled through the bottom cavity as exhaust gas
This technology is lower weight and less elaborate than current radiation shielded-propulsion systems. Equipping nuclear spacecraft systems with the NTAC Augmented Nuclear Electric Propulsion and/or Nuclear Thermal Propulsion design could reduce travel time to Mars by 50%. It has lower radar signatures compared to solar panels and could be used for systems that need to fly undetected, such as Earth observing satellites. Additionally, it has a lower noise output, which could have applications in reducing noise from submarine and aircraft carrier power systems.

Smooth-Walled Feed Horn
The technology is a monotonically-profiled, smooth-walled scalar feed horn optimized between 33 and 45 GHz. The phase center for this horn is near the aperture and is stable in frequency. The feed horns monotonic profile is compatible with machining by progressive plunge milling in which successively more accurate tools are used to realize the feed profile. This technique has been used for individual feeds and is potentially useful for fabricating large arrays of feed horns, such as multimode Winston concentrators, direct-machined smooth-walled conical feed horns, and for dual-mode feed horns.

Low-Power Charged Particle Detector
Conventional scintillation radiation detectors make use of materials that emit light when hit by ionizing radiation. These large, bulky scintillators range anywhere from 6 inches to 6 feet in size. They are attached to glass photomultiplier tubes (PMTs), which are fragile, require high voltages, and are extremely sensitive to temperature changes. Conventional detectors also include a wave shifter (a material with a dopant to re-emit the scintillator light to match the sensitivity of the PMT or photodiode) which reduces efficiency and introduces unwanted weight and bulk. Glenn's particle counter overcomes these challenges. A prototype was constructed from off-the-shelf components, and features a small scintillator (less than six inches long) and a low-voltage, UV-sensitive, wide-bandgap photodiode as the detector. Careful matching of the properties of the light emitted from the scintillator, and tailoring the sensitivity of the photodiode eliminates the need for a PMT and a wave shifter, not only saving space, weight, and power but also eliminating potential failure modes. By using wide-bandgap detectors, it can operate in changing temperature, vibration, pressure, and gravity conditions without need for a temperature compensation system. Built from solid-state components, Glenn's device is compact and robust.

Atomic Number (Z)-Grade Radiation Shields from Fiber Metal Laminates
This technology is a flexible, lighter weight radiation shield made from hybrid carbon/metal fabric and based on the Z-grading method of layering metal materials of differing atomic numbers to provide radiation protection for protons, electrons, and x-rays. To create this material, a high density metal is plasma spray-coated to carbon fiber. Another metal with less density is then plasma spray-coated, followed by another, and so on, until the material with the appropriate shielding properties is formed. Resins can be added to the material to provide structural adhesion, reducing the need for mechanical bonding. This material is amenable to molding and could be used to build custom radiation shielding to protect cabling and electronics in situations where traditional metal shielding is difficult to place.

Annular Ion Engine
The advantages displayed by Glenn's AIE stem from a number of novel design concepts, centered on an annular discharge chamber with a set of annular ion optics. The annular discharge chamber increases the effective anode surface area for electron collection as compared to a conventional cylindrically shaped ion thruster of equivalent beam area. With this increased surface area, the AIE can operate at higher discharge currents and therefore high beam currents, thereby yielding a significantly increased (3x) thrust density. An annular-geometry flat electrode can be added to enable higher-perveance designs with even higher thrust densities, with improved F/P and efficiencies compared to more conventional, spherically domed electrodes. In addition, Glenn's design allows the neutralizer cathode assembly (NCA) to be placed in a central position within the annulus, which not only eliminates the cantilevered-outboard NCA used in most conventional ion thrusters but also enables a shared gimbal platform. These benefits make manufacturing the AIE simpler as well as allowing more compact engine designs. All of these advantages add up to an electric propulsion machine that yields superior performance over the entire Isp range, making the AIE attractive for next-generation SEP vehicles. Glenn's technological advance enables spacecraft to travel farther, faster, and more cheaply than with any other propulsion technology - with clear benefits for NASA and commercial space applications.