Search
aerospace
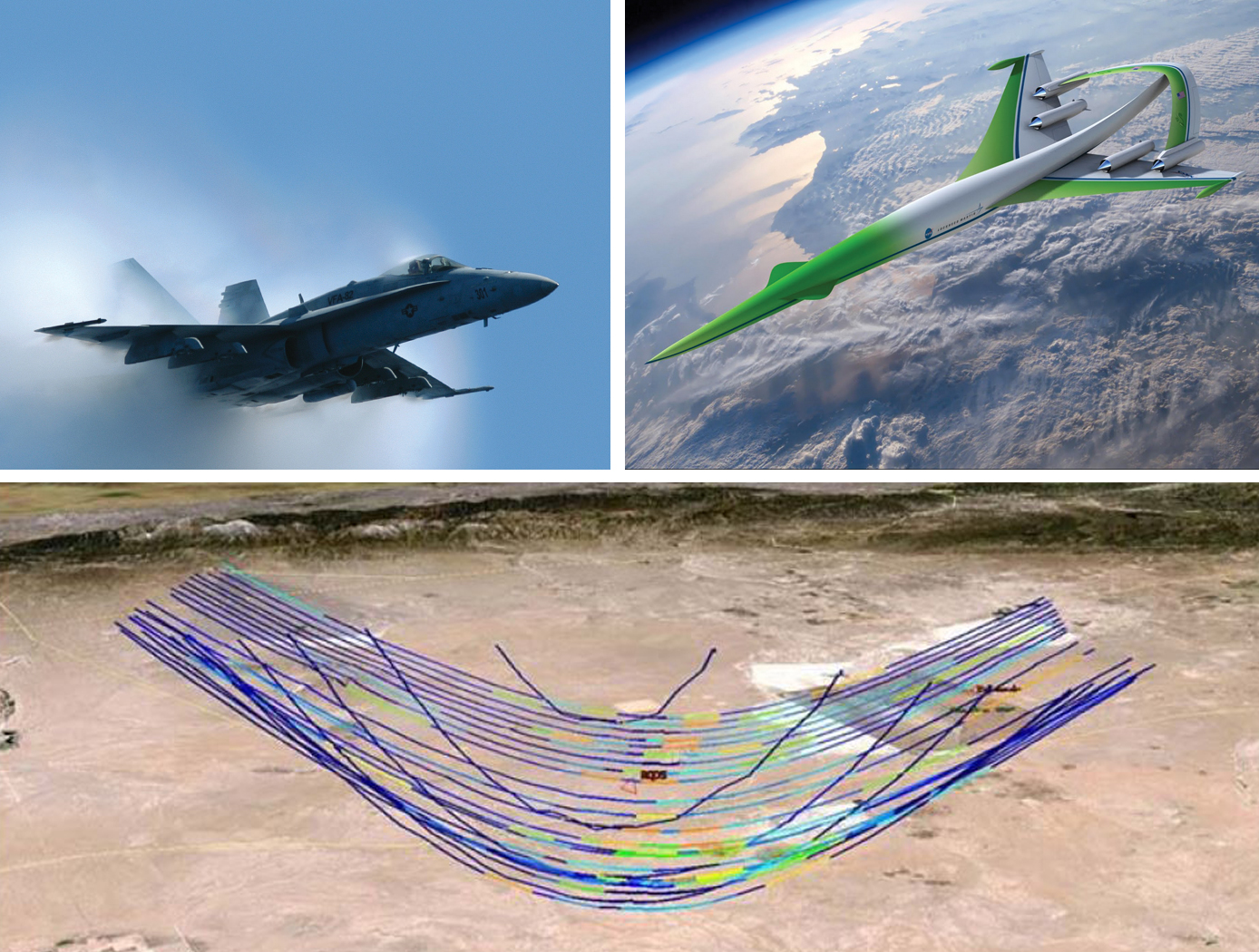
Interactive Sonic Boom Display
A supersonic shock wave forms a cone of pressurized air molecules that propagates outward in all directions and extends to the ground. Factors that influence sonic booms include aircraft weight, size, and shape, in addition to its altitude, speed, acceleration and flight path, and weather or atmospheric conditions. NASA's Real-Time Sonic Boom Display takes all these factors into account and enables pilots to control and mitigate sonic boom impacts.
<strong>How It Works</strong>
Armstrong's technology incorporates 3-dimensional (3D) Earth modeling and inputs of 3D atmospheric data. Central to the innovation is a processor that calculates significant information related to the potential for sonic booms based on an aircraft's specific operation. The processor calculates the sonic boom near a field source based on aircraft flight parameters, then ray traces the sonic boom to a ground location taking into account the near field source, environmental condition data, terrain data, and aircraft information. The processor signature ages the ray trace information to obtain a ground boom footprint and also calculates the ray trace information to obtain Mach cutoff condition altitudes and airspeeds.
Prediction data are integrated with a real-time, local-area moving-map display that is capable of displaying the aircraft's currently generated sonic boom footprint at all times. A pilot can choose from a menu of pre-programmed maneuvers such as accelerations, turns, or pushovers and the predicted sonic boom footprint for that maneuver appears on the map display. This allows pilots to select or modify a flight path or parameters to either avoid generating a sonic boom or to place the sonic boom in a specific location. The system also provides pilots with guidance on how to execute a chosen maneuver.
<strong>Why It Is Better</strong>
No other system exists to manage sonic booms in-flight. NASA's approach is unique in its ability to display in real time the location and intensity of shock waves caused by supersonic aircraft. The system allows pilots to make in-flight adjustments to control the intensity and location of sonic booms via an interactive display that can be integrated into cockpits or flight control rooms. The technology has been in use in Armstrong control rooms and simulators since 2000 and has aided several sonic boom research projects.
Aerospace companies have the technological capability to build faster aircraft for overland travel; however, the industry has not yet developed a system to support flight planning and management of sonic booms. The Real-Time Sonic Boom Display fills this need. The capabilities of this cutting-edge technology will help pave the way toward overland supersonic flight, as it is the key to ensuring that speed increases can be accomplished without disturbing population centers.
optics
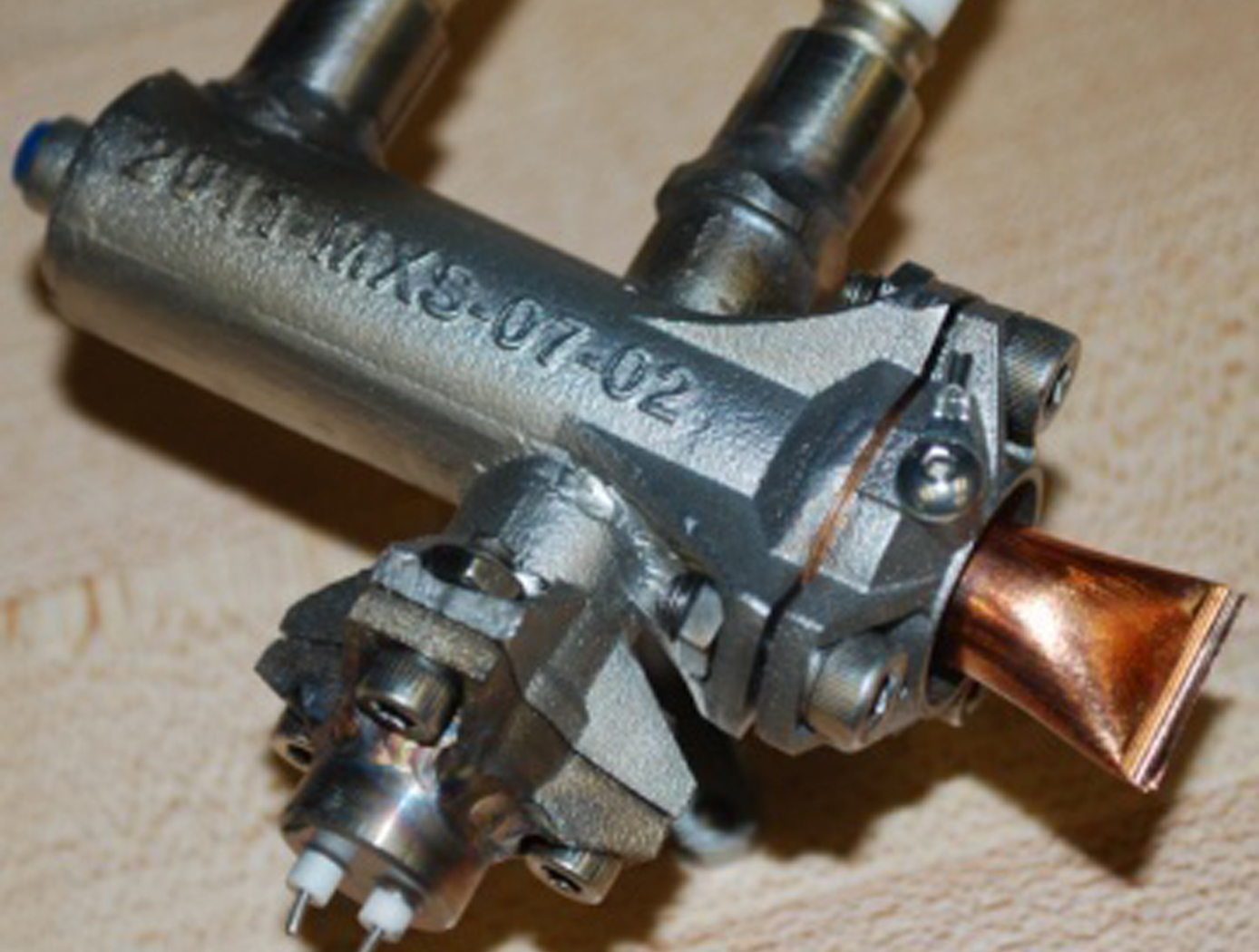
Miniaturized High-Speed Modulated X-Ray Source (MXS)
The MXS produces electrons by shining UV light from an LED onto a photocathode material such as magnesium. The electrons are then accelerated across several kV and into a chosen target material; deceleration produces X-rays characteristic of the target. The MXS uses an electron multiplier for high X-ray production efficiency.
The MXS is more compact, rugged, and power-efficient than standard X-ray sources. It can be manufactured using commercially available components and 3D printed housing, resulting in a low cost to manufacture. Unlike traditional X-ray sources, the MXS does not require a filament or vacuum and cooling systems. Most importantly, enabling rapid and arbitrary modulation allows using X-rays in the time domain, a new dimension to X-ray applications.
aerospace
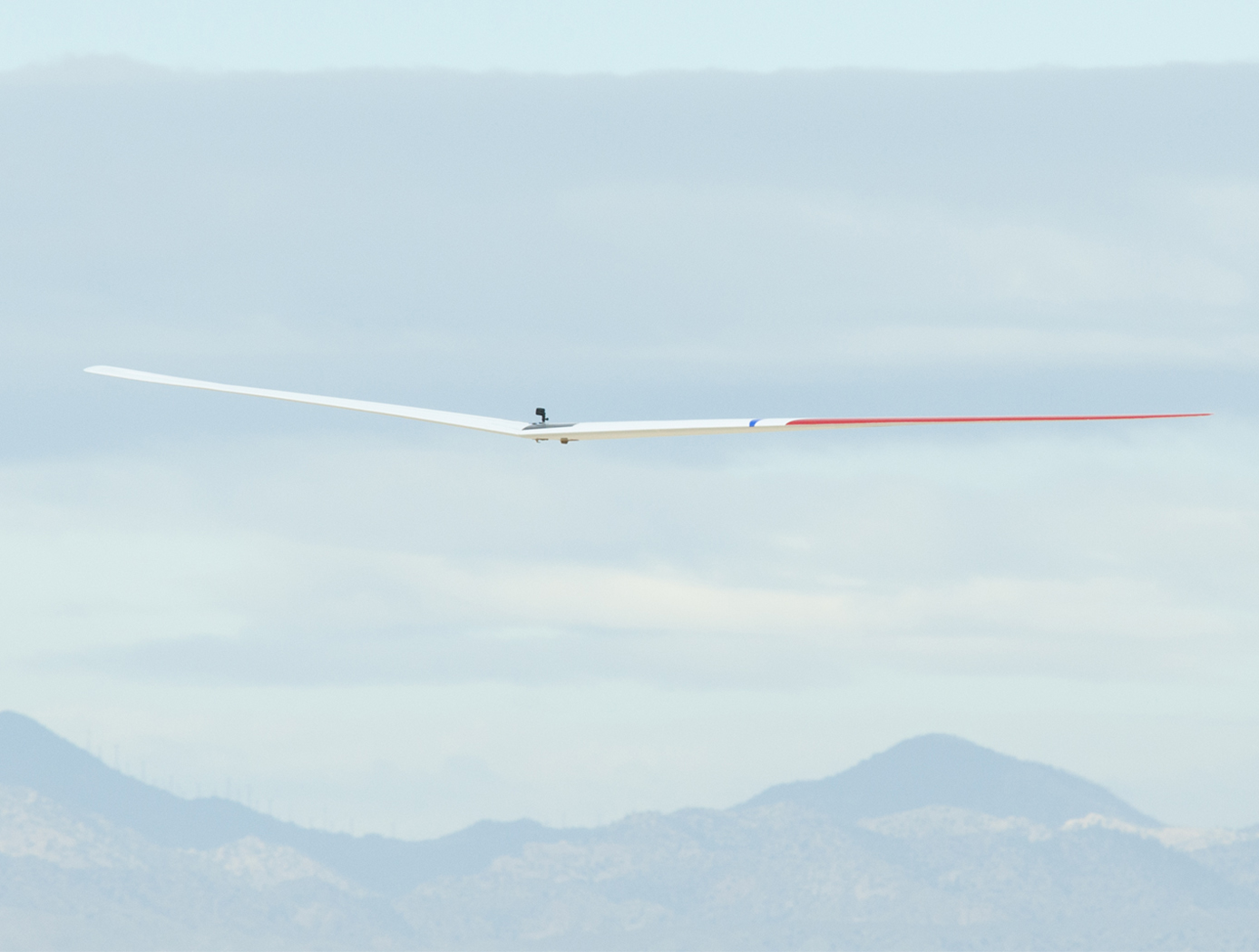
New Wing Design Exponentially Increases Total Aircraft Efficiency
Adverse yaw, present in current aircraft design, is the adverse horizontal movement around a vertical axis of an aircraft; the yaw opposes the direction of a turn. As an aircraft turns, differential drag of the left and right wings while banking contributes to aircraft yaw. Proverse yaw—yawing in the same direction as a turn—would optimize aircraft performance. Initial results from flight experiments at Armstrong demonstrated that this wing design unequivocally established proverse yaw. This wing design further reduces drag due to lift at the same time.
<strong>How It Works</strong>
The Armstrong team (supported by a large contingent of NASA Aeronautics Academy interns) built upon the 1933 research of the German engineer Ludwig Prandtl to design and validate a scale model of a non-elliptical loaded wing that reduces drag and increases efficiency.
The key to the innovation is reducing the drag of the wing through use of an alternative bell-shaped spanload, as opposed to the conventional elliptical spanload. To achieve the bell spanload, designers used a sharply tapered wing, with 12 percent less wing area than the comparable elliptical spanload wing. The new wing has 22 percent more span and 11 percent less area, resulting in an immediate 12 percent drag reduction.
Furthermore, using twist to achieve the bell spanload produces induced thrust at the wing tips, and this forward thrust increases when lift is increased at the wingtips for roll control. The result is that the aircraft rolls and yaws in the same direction as a turn, eliminating the need for a vertical tail. When combined with a blended-wing body, this approach maximizes aerodynamic performance, minimizes weight, and optimizes flight control.
<strong>Why It Is Better</strong>
Conventional aircraft make use of elliptical loaded wings to minimize drag. However, achieving aircraft stability and control in conventional elliptical wings produces a strong adverse yaw component in roll control (i.e., the aircraft will yaw the opposite direction with application of roll control). Therefore, a vertical tail or some other method of direct yaw control is required, such as split elevons for use as drag rudders. The use of elliptical wings also results in a suboptimal amount of structure to carry the integrated wing bending moment.
Adopting the bell-shaped spanload change results in an immediate 12 percent drag reduction. In addition, optimization of the overall aircraft configuration is projected to achieve additional significant overall performance increases.
information technology and software

Traffic Aware Strategic Aircrew Requests (TASAR)
The NASA software application developed under the TASAR project is called the Traffic Aware Planner (TAP). TAP automatically monitors for flight optimization opportunities in the form of lateral and/or vertical trajectory changes. Surveillance data of nearby aircraft, using ADS-B IN technology, are processed to evaluate and avoid possible conflicts resulting from requested changes in the trajectory. TAP also leverages real-time connectivity to external information sources, if available, of operational data relating to winds, weather, restricted airspace, etc., to produce the most acceptable and beneficial trajectory-change solutions available at the time. The software application is designed for installation on low-cost Electronic Flight Bags that provide read-only access to avionics data. The user interface is also compatible with the popular iPad. FAA certification and operational approval requirements are expected to be minimal for this non-safety-critical flight-efficiency application, reducing implementation cost and accelerating adoption by the airspace user community.
Awarded "2016 NASA Software of the Year"
aerospace
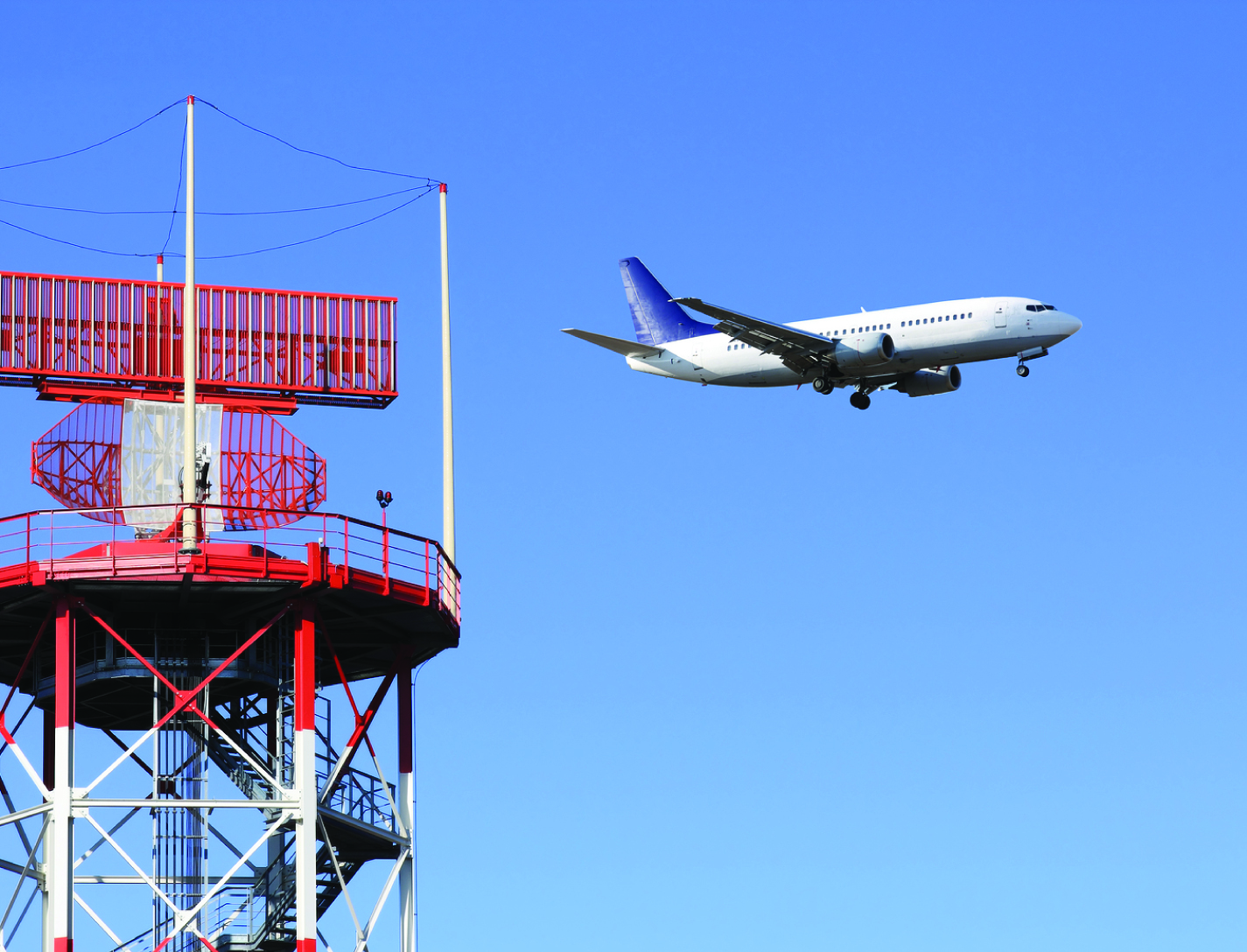
Dynamic Weather Routes Tool
Every 12 seconds, the Dynamic Weather Route (DWR) automation system computes and analyzes trajectories for en-route flights. DWR first identifies flights that could save 5 or more flying minutes (wind-corrected) by flying direct to a downstream return fix on their current flight plan. Eligible return fixes are limited so as not to take flights too far off their current route or interfere with arrival routings near the destination airport. Using the direct route as a reference route, DWR inserts up to two auxiliary waypoints as needed to find a minimum-delay reroute that avoids the weather and returns the flight to its planned route at the downstream fix. If a reroute is found that can save 5 minutes or more relative to the current flight plan, the flight is posted to a list displayed to the airline or FAA user. Auxiliary waypoints are defined using fix-radial-distance format, and a snap to nearby named fix option is available for todays voice-based communications. Users may also adjust the alert criteria, nominally set to 5 minutes, based on their workload and desired potential savings for their flights. A graphical user interface enables visualization of proposed routes on a traffic display and modification, if necessary, using point, click, and drag inputs. If needed, users can adjust the reroute parameters including the downstream return fix, any inserted auxiliary waypoints, and the maneuver start point. Reroute metrics, including flying time savings (or delay) relative to the current flight plan, proximity to current and forecast weather, downstream sector congestion, traffic conflicts, and conflicts with special use airspace are all updated dynamically as the user modifies a proposed route.
aerospace
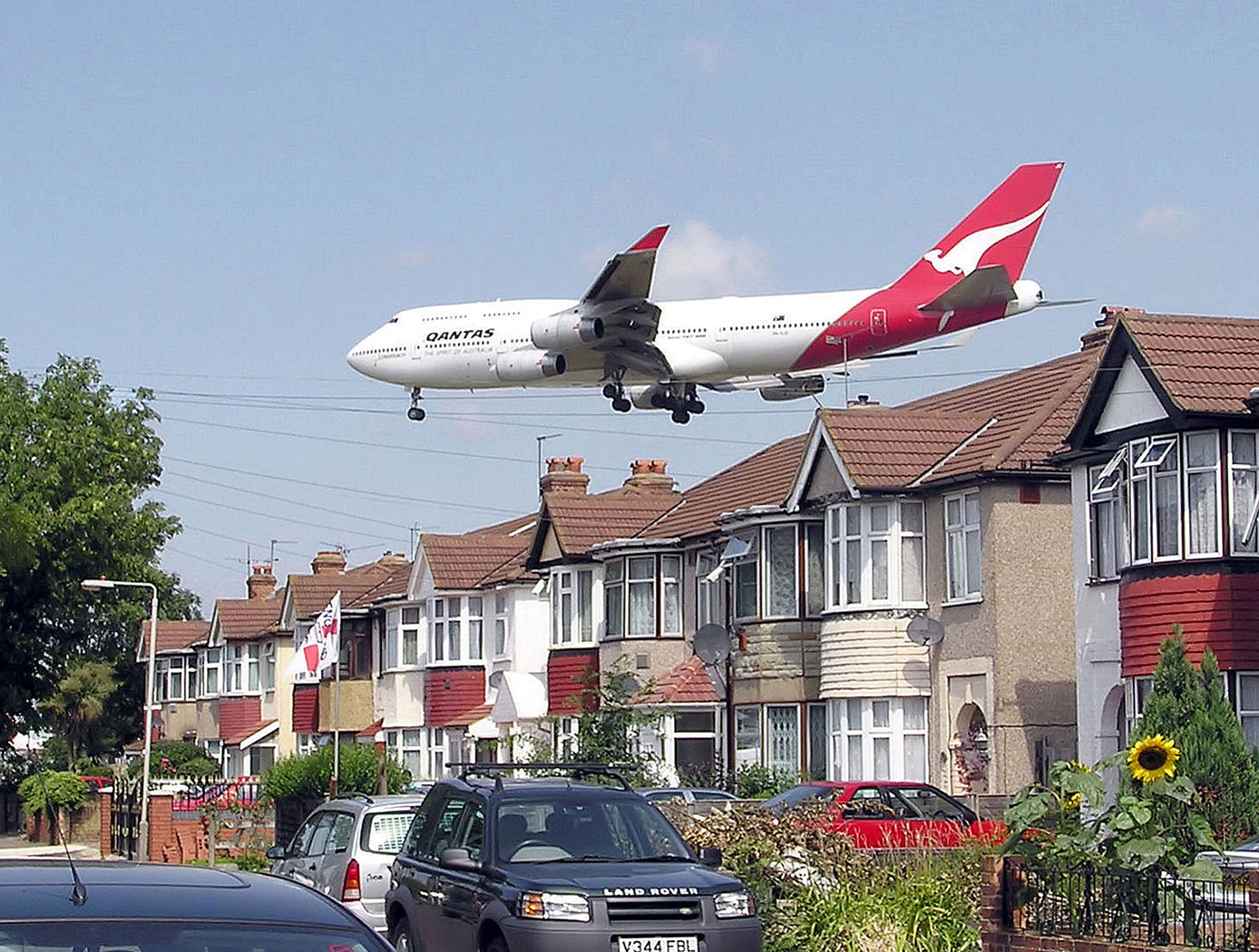
Compact, Lightweight, CMC-Based Acoustic Liner
NASA researchers are extending an existing oxide/oxide CMC sandwich structure concept that provides mono-tonal noise reduction. That oxide/oxide CMC has a density of about 2.8 g/cc versus the 8.4 g/cc density of a metallic liner made of IN625, thus offering the potential for component weight reduction. The composites have good high-temperature strength and oxidation resistance, allowing them to perform as core liners at temperatures up to 1000°C (1832°F). NASA's innovation uses cells of different lengths or effective lengths within a compact CMC-based liner to achieve broadband noise reduction. NASA has been able to optimize the performance of the proposed acoustic liner by using improved design tools that help reduce noise over a specified frequency range. One such improvement stems from the enhanced understanding of variable-depth liners, including the benefits of alternate channel shapes/designs (curved, bent, etc.). These new designs have opened the door for CMC-based acoustic liners to offer core engine noise reduction in a lighter, more compact package. As a first step toward demonstrating advanced concepts, an oxide/oxide CMC acoustic testing article with different channel lengths was tested. Bulk absorbers could also be used, either in conjunction with or in place of the liners internal chambers, to reduce noise further if desired.
aerospace
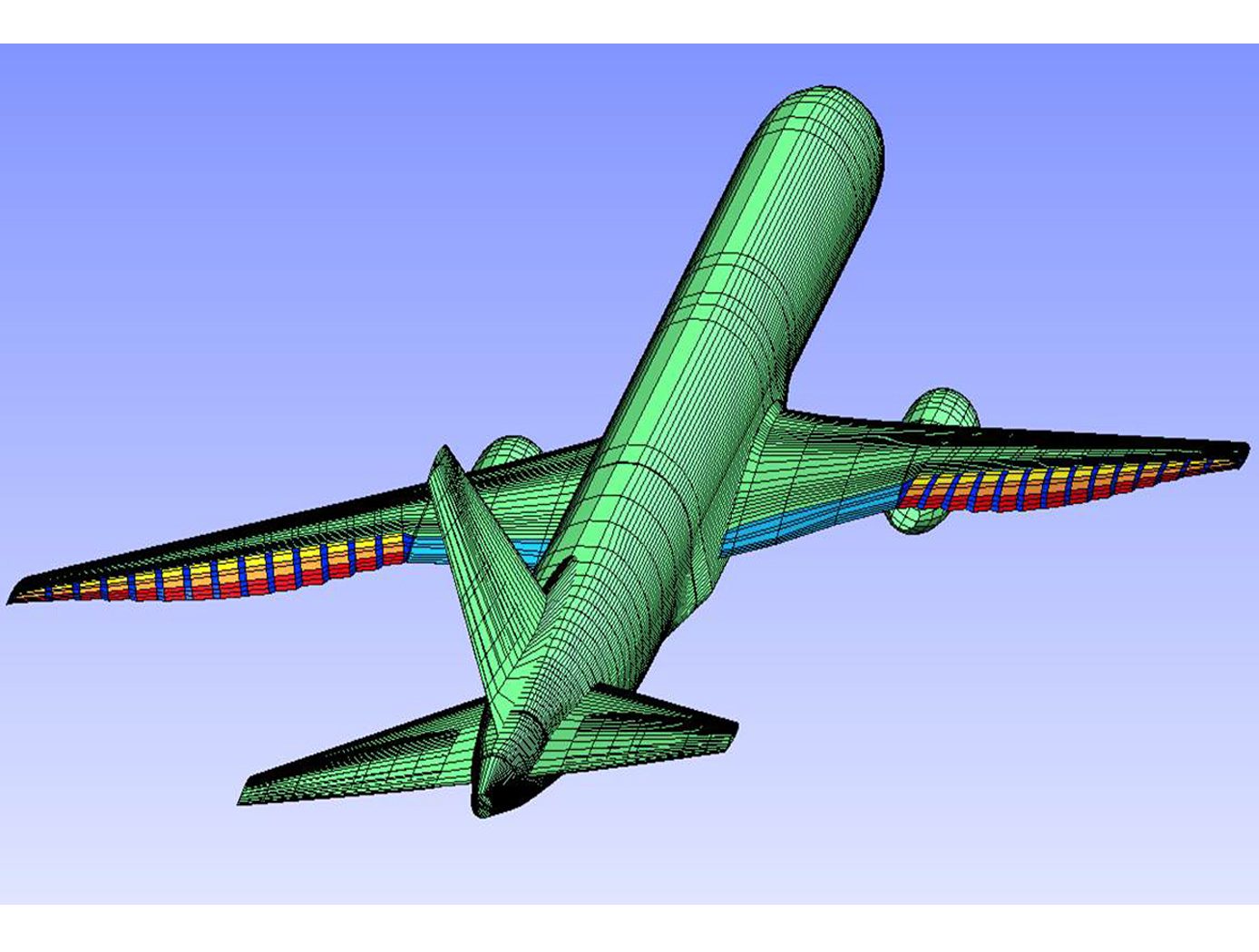
Aeroelastic Wing Shaping
Distributed propulsion and lightweight flexible structures on air vehicles pose a significant opportunity to improve mission performance while meeting next generation requirements including reduced fuel burn, lower emissions, and enhanced takeoff and landing performance. Flexible wing-shaping aircraft using distributed propulsion enable the ability to achieve improved aerodynamic efficiency while maintaining aeroelastic stability. Wing shaping concepts using distributed propulsion leverage the ability to introduce forces/ moments into the wing structure to affect the wing aerodynamics. This can be performed throughout the flight envelope to alter wing twist, hence local angle of attack, as the wing loading changes with air vehicle weight during cruise. Thrust-induced lift can be achieved by distributed propulsion for enhanced lift during take-off and landing. For a highly flexible wing structure, this concept could achieve a 4% improvement in lift-to-drag ratio, hence reduced fuel burn, as compared to a conventional stiff wing. This benefit is attributed to a reduction in lift-induced drag throughout the flight envelope by actively shaping the spanwise lift distribution using distributed propulsion. Vertical tail size could be reduced by utilizing differential thrust flight-propulsion control. This will result in weight reduction to achieve further fuel savings. Aeroelastic stability is addressed in the design process to meet flutter clearance requirements by proper placement of the propulsion units. This technology enables synergistic interactions between lightweight materials, propulsion, flight control, and active aeroelastic wing shaping control for reducing the environmental impact of future air vehicles.
aerospace
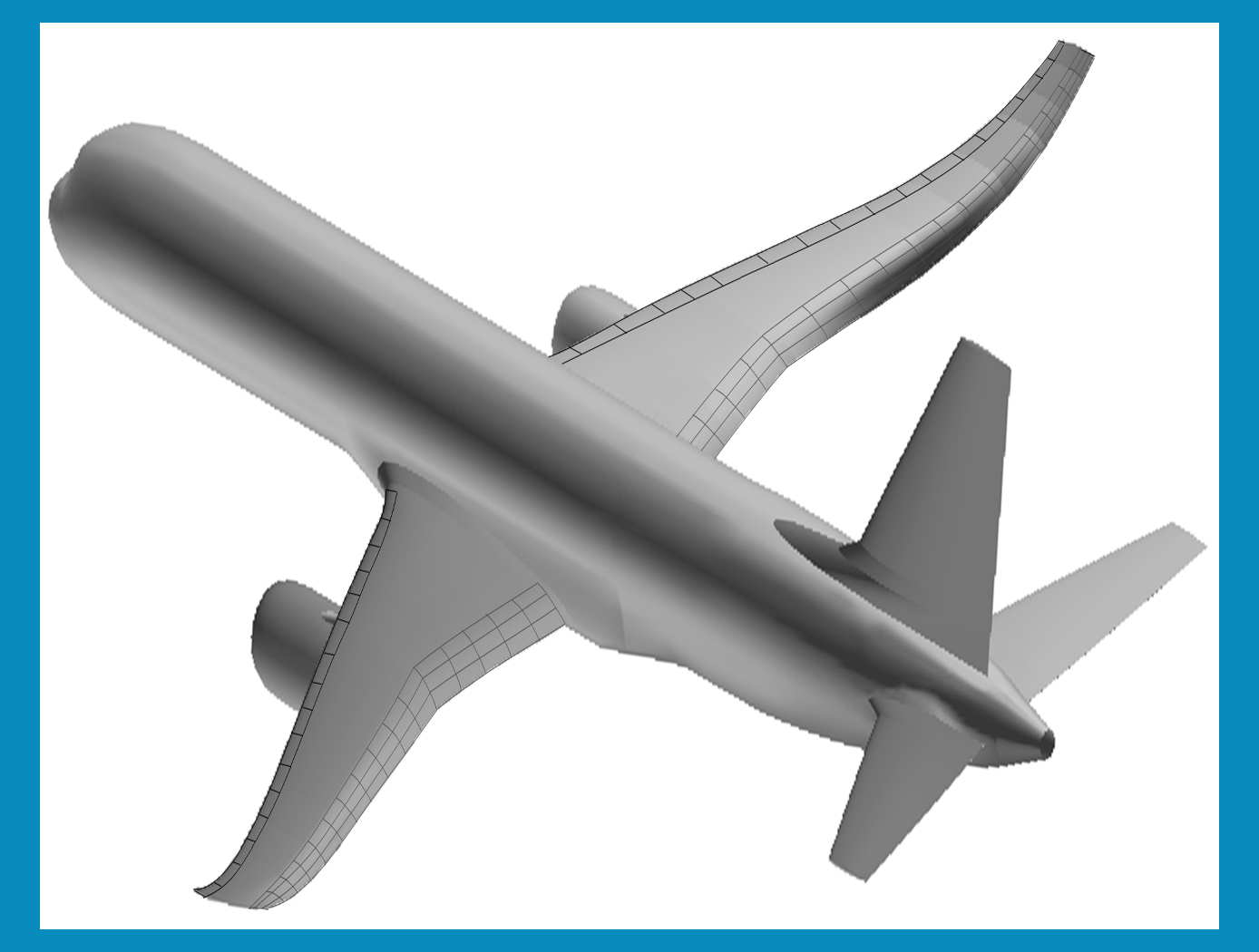
Green aviation - improved aerodynamic efficiency and less fuel burn
Currently, as fuel is burned, wing loading is reduced, thereby causing the wing shape to bend and twist. This wing-shape change causes the wings to be less aerodynamically efficient. This problem can be further exacerbated by modern high-aspect flexible wing design. Aircraft designers typically address the fuel efficiency goal by reducing aircraft weights, improving propulsion efficiency, and/or improving the aerodynamics of aircraft wings passively. In so doing, the potential drag penalty due to changes in the wing shapes still exists at off-design conditions. The unique or novel features of the new concepts are:
1. Variable camber flap provides the same lift capability for lower drag as compared to a conventional flap. The variable camber trailing edge flap (or leading edge slat) comprises multiple chord-wise segments (three or more) to form a cambered flap surface, and multiple span-wise segments to form a continuous trailing edge (or leading edge) curve with no gaps which could be prescribed by a mathematical function or the equivalent with boundary conditions enforced at the end points to minimize tip vortices
2. Continuous trailing edge flap (or leading edge slat) provides a continuously curved trailing edge (or leading edge) with no gaps to minimize vortices that can lead to an increase in drag.
3. The active wing-shaping control method utilizes the novel flap (or slat) concept described herein to change a wing shape to improve aerodynamic efficiency by optimizing span-wise aerodynamics.
4. An aeroelastic wing shaping method for analyzing wing deflection shape under aerodynamic loading is used in a wing-control algorithm to compute a desired command for the flap-actuation system to drive the present flap (or slat) system to the correct position for wing shaping.
aerospace
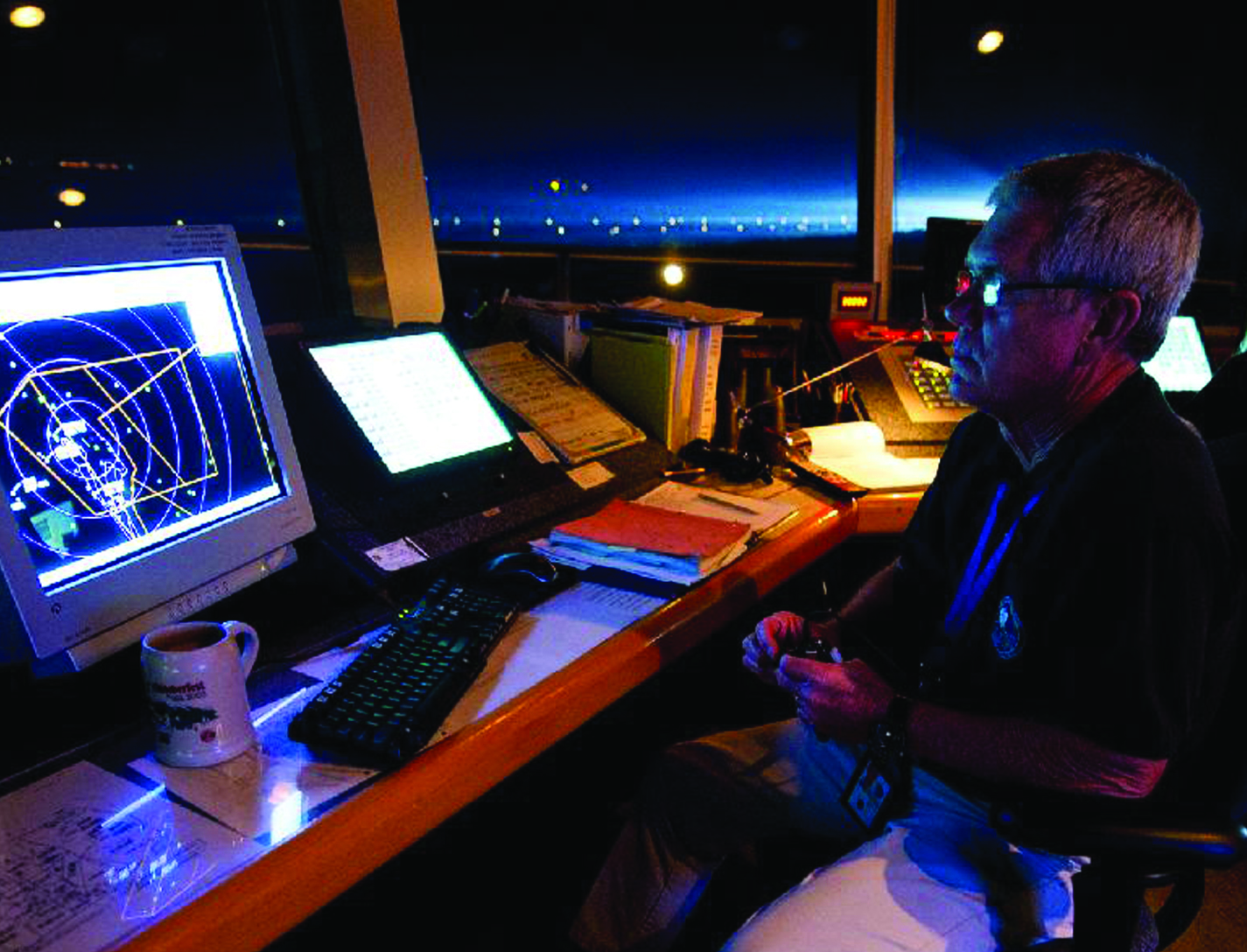
FACET: Future Air Traffic Management Concepts Evaluation Tool
Actual air traffic data and weather information are utilized to evaluate an aircrafts flight-plan route and predict its trajectories for the climb, cruise, and descent phases. The dynamics for heading (the direction the aircraft nose is pointing) and airspeed are also modeled by the FACET software, while performance parameters, such as climb/descent rates and speeds and cruise speeds, can also be obtained from data tables. The resulting trajectories and traffic flow data are presented in a 3-D graphical user interface. The FACET software is modular and is written in the Java and C programming languages. Notable FACET applications include reroute conformance monitoring algorithms that have been implemented in one of the Federal Aviation Administrations nationally deployed, real-time operational systems.
aerospace

Co-Optimization of Blunt Body Shapes for Moving Vehicles
Vehicles designed for purposes of exploration of the planets and other atmospheric bodies in the Solar System favor the use of mid-Lift/Drag blunt body geometries. Such shapes can be designed so as to yield favorable hypersonic aerothermodynamic properties for low heating and hypersonic aerodynamic properties for maneuverability and stability. The entry trajectory selected influences entry peak heating and integrated heating loads which in turn influences the design of the thermal protection system. A nominal is used to compare each shape considered. The vehicle will be subject to both launch and entry loading along with structural integrity constraints that may further influence shape design. Further, such vehicles must be sized so as to fit on existing or realizable launch vehicles, often within existing launch payload shroud constraints.