Miniaturized High-Speed Modulated X-Ray Source (MXS)
optics
Miniaturized High-Speed Modulated X-Ray Source (MXS) (GSC-TOPS-51)
Small, Low-Cost Option for High-Speed Modulation of X-Ray Intensity
Overview
This miniaturized X-ray source can be modulated in intensity from completely off to full intensity, over 100 keV, in subnanosecond timescales. The high speed switching capability and miniature size make possible many new technologies including X-ray-based communication, compact time resolved X-ray diffraction, novel X-ray fluorescence instruments, low precise dose medical X-rays, and more.
The Technology
The MXS produces electrons by shining UV light from an LED onto a photocathode material such as magnesium. The electrons are then accelerated across several kV and into a chosen target material; deceleration produces X-rays characteristic of the target. The MXS uses an electron multiplier for high X-ray production efficiency.
The MXS is more compact, rugged, and power-efficient than standard X-ray sources. It can be manufactured using commercially available components and 3D printed housing, resulting in a low cost to manufacture. Unlike traditional X-ray sources, the MXS does not require a filament or vacuum and cooling systems. Most importantly, enabling rapid and arbitrary modulation allows using X-rays in the time domain, a new dimension to X-ray applications.
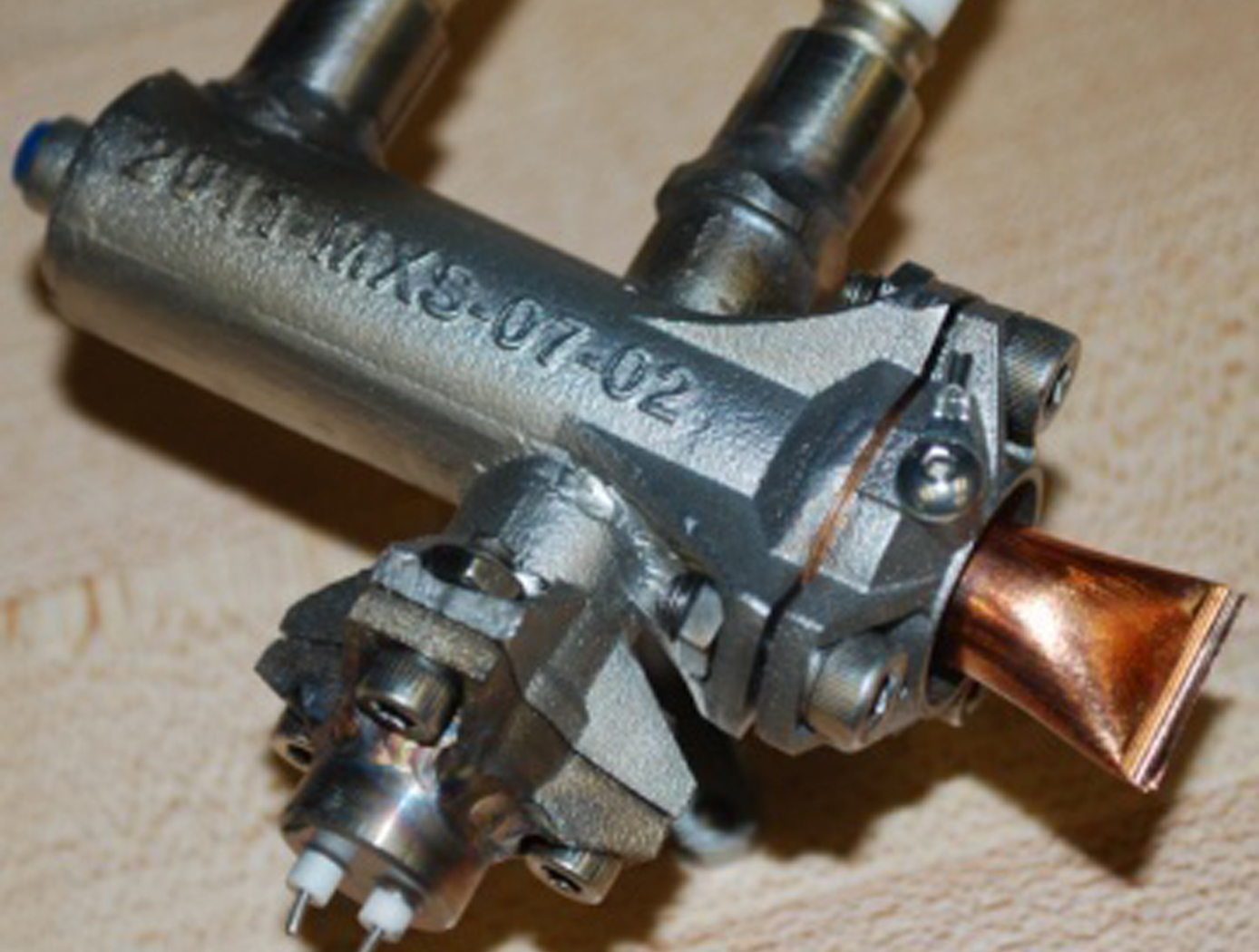
Benefits
- Arbitrary modulation from 0 to 100+ keV within a nanosecond
- Small size: Weighs around 160 g
- Low cost to produce
- Energy efficient
- Rugged: No filament burnout and no vacuum or cooling systems required
- Enables X-ray communication
- Secure, fast, and long-range
- More power efficient than current and next-gen laser-based space communication
- Works with hypersonic aircraft in atmosphere
Applications
- Secure, power-efficient X-ray-based communications
- In-flight calibration of X-ray detectors
- Compact, time-resolved X-ray diffraction and fluorescence
- Precise- and low-dose medical X-ray imaging
- Chemical/material analysis, resource identification (e.g., mining), and nondestructive testing (e.g., metal fatigue)
Similar Results

Low-Power Charged Particle Detector
Conventional scintillation radiation detectors make use of materials that emit light when hit by ionizing radiation. These large, bulky scintillators range anywhere from 6 inches to 6 feet in size. They are attached to glass photomultiplier tubes (PMTs), which are fragile, require high voltages, and are extremely sensitive to temperature changes. Conventional detectors also include a wave shifter (a material with a dopant to re-emit the scintillator light to match the sensitivity of the PMT or photodiode) which reduces efficiency and introduces unwanted weight and bulk. Glenn's particle counter overcomes these challenges. A prototype was constructed from off-the-shelf components, and features a small scintillator (less than six inches long) and a low-voltage, UV-sensitive, wide-bandgap photodiode as the detector. Careful matching of the properties of the light emitted from the scintillator, and tailoring the sensitivity of the photodiode eliminates the need for a PMT and a wave shifter, not only saving space, weight, and power but also eliminating potential failure modes. By using wide-bandgap detectors, it can operate in changing temperature, vibration, pressure, and gravity conditions without need for a temperature compensation system. Built from solid-state components, Glenn's device is compact and robust.

X-Ray Diffraction Method to Detect Defects in Cubic Semiconductor (100) Wafers
This technology is a method of using x-ray diffraction (XRD) to evaluate the concentration of crystal structure defects, and thus the quality, of cubic (100)-oriented semiconductor wafers. Developed to enhance NASA's capabilities in fabricating chips for aeronautics applications, the method supplants existing methods that not only destroy the wafer in question, but can take as long as a day to determine the quality of a single wafer. The approach can be used with any commonly used semiconductor, including silicon, SiGe, GaAs and others, in a cubic (100) orientation, which covers at least 90% of commercial wafers. It can also be used to evaluate the quality of epi layers deposited on wafer substrates, and of ingots before they are sliced into wafers.

Phononic Isolated Kinetic Inductance Detector Fabrication Process
The Phononic Isolated KID Fabrication Process utilizes a silicon on insulator, or a silicon wafer coated with a thin film bilayer of silicon oxide and silicon nitride (or amorphous silicon), to be used as the starting wafer. The silicon or silicon nitride thin films act as the structural material. The films thicknesses are chosen based on the desirable phononic crystal properties. The phononic crystal is patterned with electron beam lithography to get minimum features. The structures are etched in a fluorine plasma chemistry stopping on the oxide layer. The niobium or other superconductor layer is deposited, patterned, and etched. This can be done in a liftoff process so as not to damage the SiN or Si underlayer. A hafnium or other superconductor is deposited, patterned, and etched to function as the kinetic inductance material. A silicon handle wafer is patterned and etched using deep reactive ion etching which stops on the silicon oxide. The silicon oxide is etched in hydroflouric acid and a wax bonding material used as a temporary bonding material is dissolved. The final structure is removed from the temporary handle wafer. This process is beneficial in that it is simple aside from the incorporation of the nanostructured membranes.
The Phononic Isolated KID Fabrication Process is an implementation of incorporation of a phononic crystal into a KID architecture. The process can also incorporate a second dieletric material such as nanocrystalline diamond that can be used as a stiffening material for the phononic crystal.

Sublimable Propellant Source for Iodine-fed Ion Propulsion System
NASAs iodine vapor feed system is based on a mechanism that holds and maintains the solid iodine is contact with a heated surface, in this case the walls of the propellant tank. The mechanism provides a robust and reliable steady-state delivery of sublimated iodine vapor to the ion propulsion system by ensuring good thermal contact between the solid iodine and the tank walls.
To date, the technology development effort includes extensive thermal, mechanical and flow modelling together with testing of components and subsystems required to feed iodine propellant to a 200-W Hall thruster. The feed system has been designed to use materials that are resistant to the highly-reactive nature of iodine propellant. Dynamic modeling indicates that the feed system tubing can be built is such a way as to reduce vibrationally-induced stresses that occur during launch. Thermal modeling has been performed to demonstrate that the feed system heater power levels are sufficient to heat the tank and propellant lines to operating temperatures, and sublime the iodine in the storage tank to supply propellant for reliable and long-term operation.

Fast Optical Shutter, Chopper, Modulator, and Deflector
A laser or a light source is incident on a detector. It usually passes through a shutter that can open and then close to limit the amount of light hitting the detector. There are limitations on the speed, size and cost of such apertures. The design of this technology uses DLP mirror technology. It can rapidly deflect the incoming light beam onto an aperture, which blocks the beam path, or through the aperture, which allows it to go onto the detector. The DLP mirror in this shutter uses an aperture design that is nearly 3 orders of magnitudes faster (shorter exposure time) than similar-sized aperture using conventional commercial-off-the-shelf mechanical shutters and 1-2 orders of magnitude smaller and cheaper than higher-performing custom-made shutters that are used by a few labs around the world.
The DLP mirror is actuated via a computer controlled oscillator circuit. A laser beam directed to the mirror is either passed to a target detector, or diverted, based on the inputs from the circuit. In this manner, the DLP mirror / circuit can act as a fast shutter, modulator, or chopper for the light beam. One novel feature of this invention is the application of the DLP to divert a beam onto or off a detector for instrumentation systems.