Search
power generation and storage
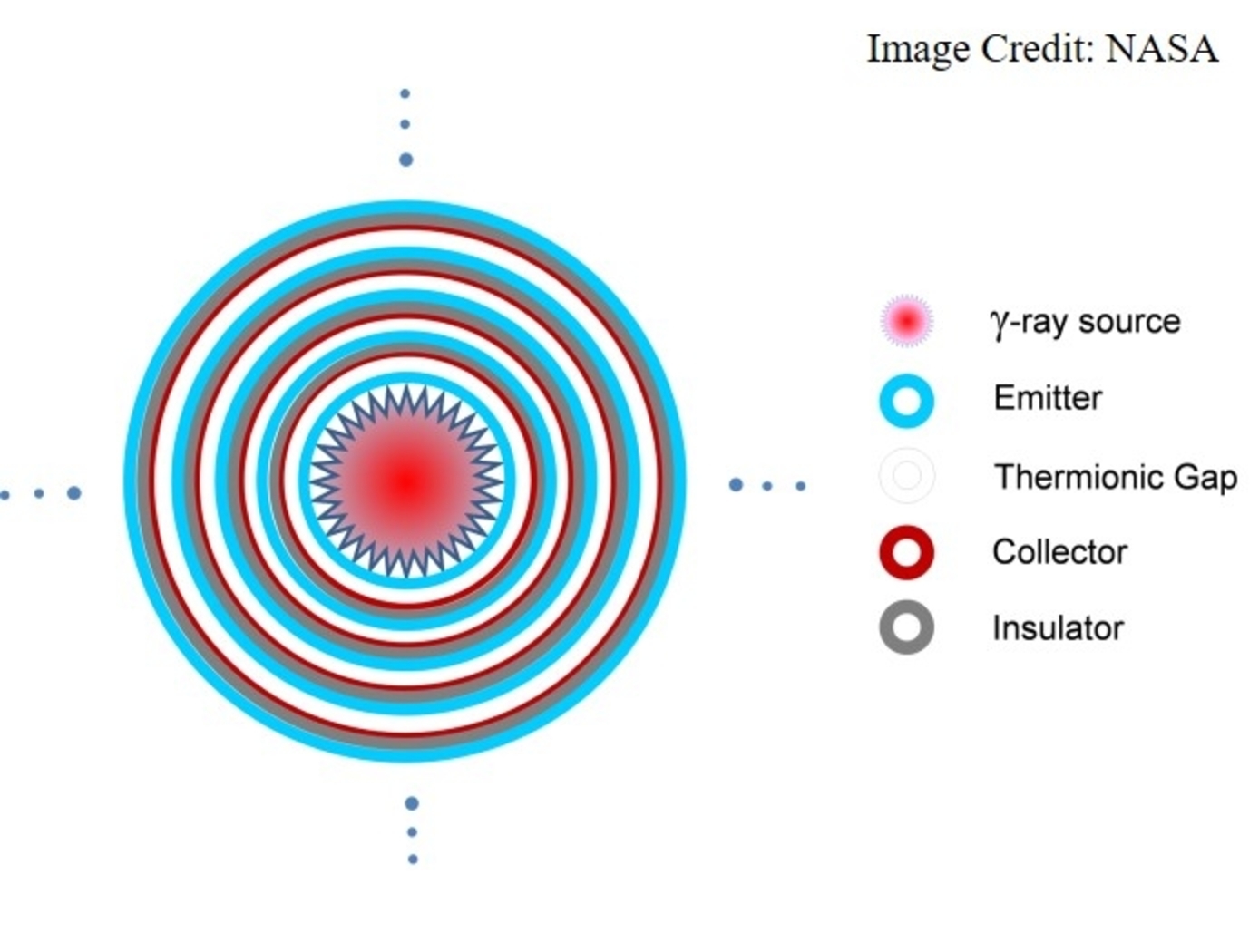
Multi-Layer Nuclear Thermionic Avalanche Cell
The Multi-Layer NTAC is comprised of a gamma-ray source and various layers of emitters, collectors, and insulators. Ideal emitter materials include elements with high atomic numbers, while ideal collector and insulator materials include elements with low atomic numbers. A high-energy gamma-ray (tens of keV to MeV) is used to liberate a large number of intra-band, inner-shell electrons from atoms within the emitter material for power generation through the primary interactions of photoelectric, Compton scattering, photonuclear, and electron/positron pair production processes. Secondary and tertiary electrons are liberated in the avalanche process as well. If a power conversion process effectively utilizes all liberated electrons in an avalanche mode through a power conversion circuit, the power output is drastically increased. Because power conversion is determined by the absorption rate of high energy photons, increasing power output requires either thicker collector material or a sufficient number of layer structures to capture the high energy photons, leaving no liberated electrons escaping (i.e., minimizing the leak of radioactive rays). The selection of materials, the thicknesses of the emitter, collector, and insulator, as well as the number of NTAC layers required are all determined by the energy of photon source. The thermal energy from radioactive decay can also be converted to electricity using a thermoelectric device to further increase power output. The Multi-Layer NTAC technology can be manufactured using existing semiconductor fabrication technology and can be tailored for small-to-large scale power needs, including kilowatt and megawatt applications.
manufacturing
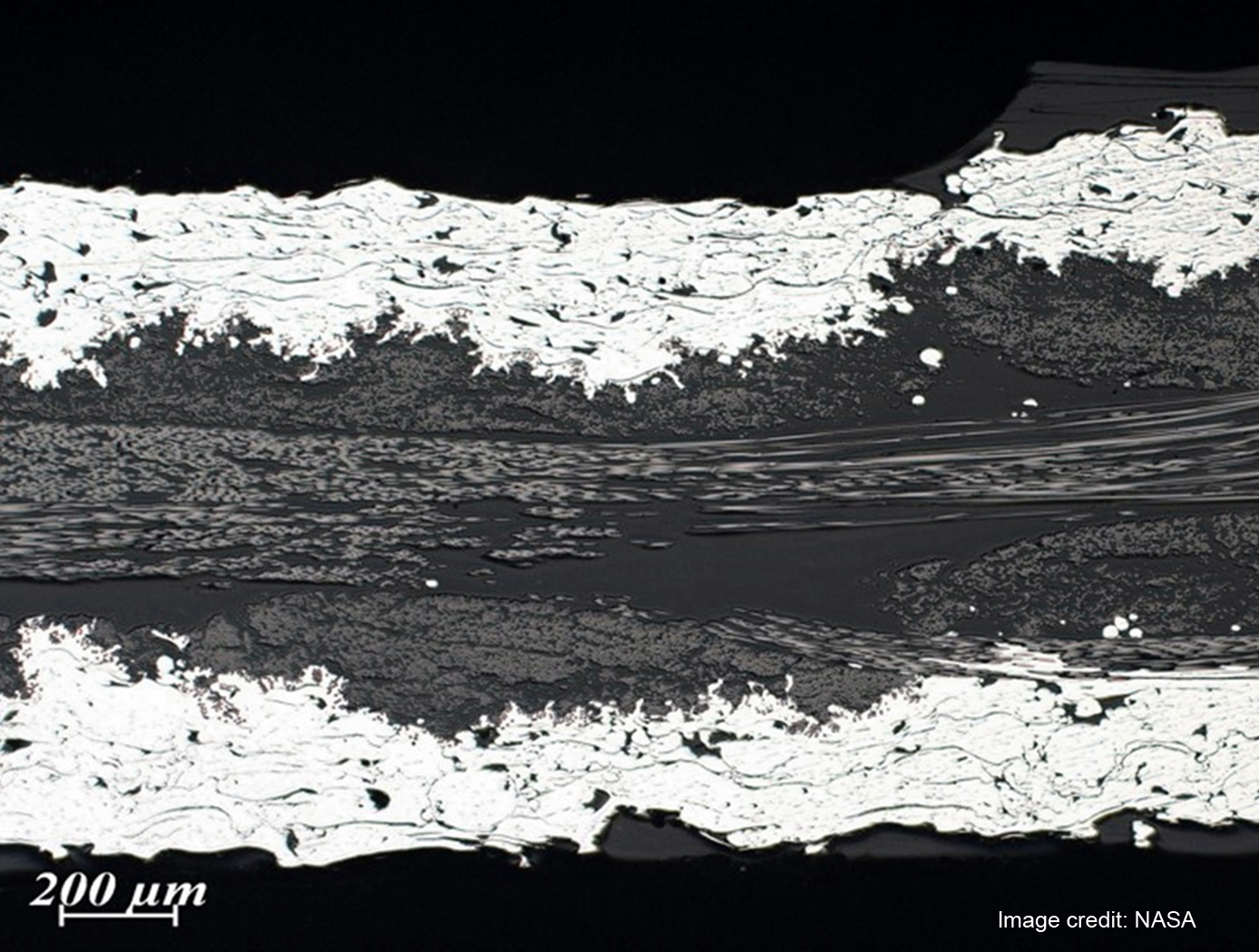
Fiber-Metal Laminate Manufacturing Technique
Fiber-Metal Laminates (FMLs) are composite materials that consist of conventional fiber reinforced plastics with the addition of a metal component, typically a foil or mesh layer(s). The metal component offers the advantage of incorporating metal-like properties to the composite construction. While a range of potential advantages and applications have been discussed for FMLs, the primary application to date has been for aircraft structures, with one potential advantage being the lightning strike protection (LSP) offered by the improved electrical conductivity. As aircraft construction has moved to composite structures, there has been an increasing need for such conductive composites. Similarly, with increasing use of composites for other large structures, e.g. wind turbines, there are an increasing number of potential applications for lightning strike protection materials. Other advantages of FML are improved impact and fire resistance.
This innovation provides a method for making FML materials that incorporate nanotube reinforcement. The method involves the use of RF plasma spray to directly form and deposit nanotube materials onto fibers/fabrics, which can then be manufactured into composite structures by infiltrating the fiber with resin, and consolidating the structure via autoclave processing or via the use Vacuum Assisted Resin Transfer Molding (VARTM) composite manufacturing methods. Nanotubes incorporated into the structure in this manner can be of several types, for example boron nitride or carbon nanotubes. The objective of this innovation is to incorporate the nanotube materials in the FML in order to improve the mechanical properties.
materials and coatings
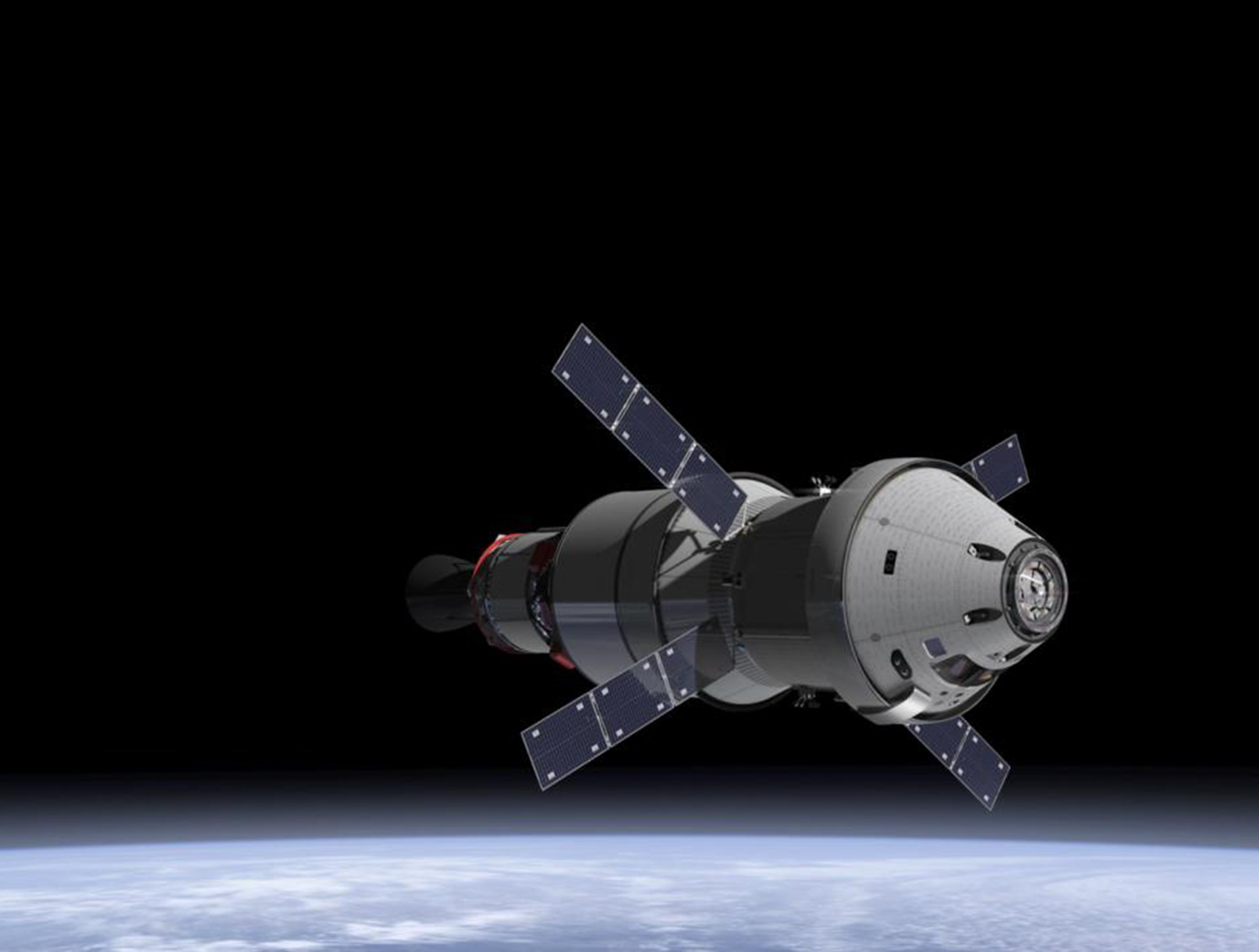
Advanced Protective Coatings for Graphite-Based Nuclear Propulsion Fuel Elements
To protect the graphite (Gr)-based substrates in a nuclear systems fuel elements from hot hydrogen attacks, earlier researchers developed a method to deposit (via chemical vapor deposition) a protective niobium carbide (NbC) or zirconium carbide (ZrC) coating in the inner cooling channels of the fuel elements through which the hydrogen propellant flows. Unfortunately, the significant difference in the coefficients of thermal expansion (CTE) between the Gr-based substrate and the ZrC coating leads to debonding at intermediate temperatures, thereby exposing the substrate to hot hydrogen attack despite the NbC or ZrC coating. Innovators at Glenn have proposed a solution to this problem by introducing additional layers of compliant metallic coatings to accommodate the differences in CTE between the ZrC and the Gr-substrate,thereby potentially increasing life and durability. In this configuration,the innermost layer is composed of molybdenum carbide (Mo<sub>2</sub>C), and additional outer layers are made of molybdenum (Mo) and niobium (Nb) layers. The MoC acts as a diffusion barrier to minimize the diffusion of carbon into the refractory metal layers and the diffusion of Mo or Nb into the Gr-based substrate. The Mo layer is deposited on top of the Mo<sub>2</sub>C layer. A Nb layer is deposited on the Mo layer with the ZrC forming the outside layer of the coating. A thin Mo layer on the ZrC helps to seal the cracks on the ZrC and acts a diffusion barrier to hydrogen diffusion into the coating.The Mo and Nb layers are compliant so that differences in the thermal expansion of ZrC and other layers can be accommodated without significant debonding or cracking. They also act as additional diffusion barriers to hydrogen diffusion towards the Gr-substrate. Overall, Glenn's pioneering use of layered coatings for these components will potentially increase the durability and performance of nuclear propulsion rockets.
materials and coatings
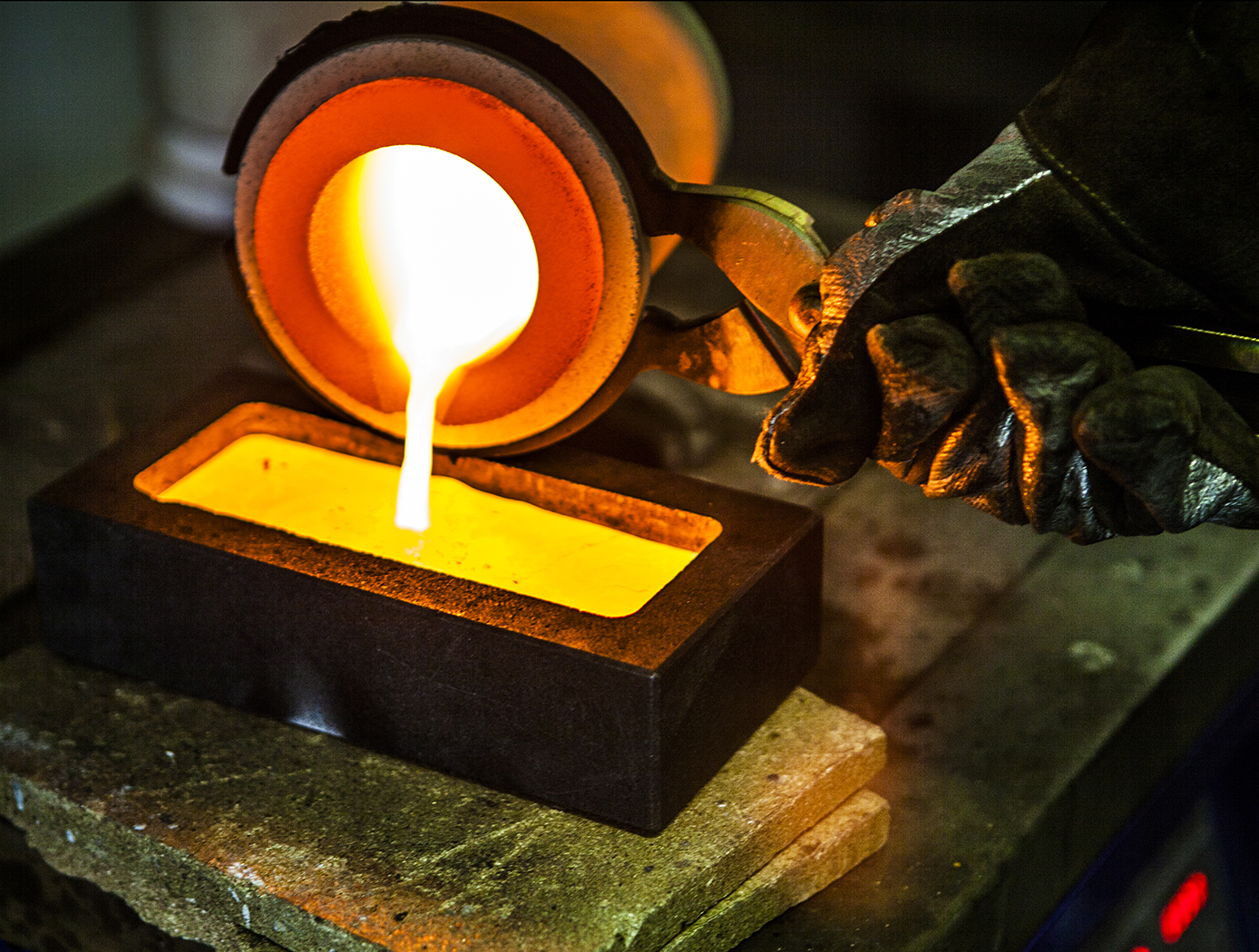
Silicon Carbide (SiC) Fiber-Reinforced SiC Matrix Composites
Aimed at structural applications up to 2700°F, NASA's patented technologies start with two types of high-strength SiC fibers that significantly enhance the thermo-structural performance of the commercially available boron-doped and sintered small-diameter “Sylramic” SiC fiber. These enhancement processes can be done on single fibers, multi-fiber tows, or component-shaped architectural preforms without any loss in fiber strength. The processes not only enhance every fiber in the preforms and relieve their weaving stresses, but also allow the preforms to be made into more shapes. Environmental resistance is also enhanced during processing by the production of a protective in-situ grown boron-nitride (iBN) coating on the fibers. Thus the two types of converted fibers are called “Sylramic-iBN” and “Super Sylramic-iBN”.
For high CMC toughness, two separate chemical vapor infiltration (CVI) steps are used, one to apply a boron nitride coating on the fibers of the preform and the other to form the SiC-based matrix. The preforms are then heat treated not only to densify and shrink the CVI BN coating away from the SiC matrix (outside debonding), but also to increase its creep resistance, temperature capability, and thermal conductivity.
One crucial advantage in this suite of technologies lies in its unprecedented customizability. The SiC/SiC CMC can be tailored to specific conditions by down-selecting the optimum fiber, fiber coating, fiber architecture, and matrix materials and processes. In any formulation, though, the NASA-processed SiC fibers display high tensile strength and the best creep-rupture resistance of any commercial SiC fiber, with strength retention to over 2700°F.
environment
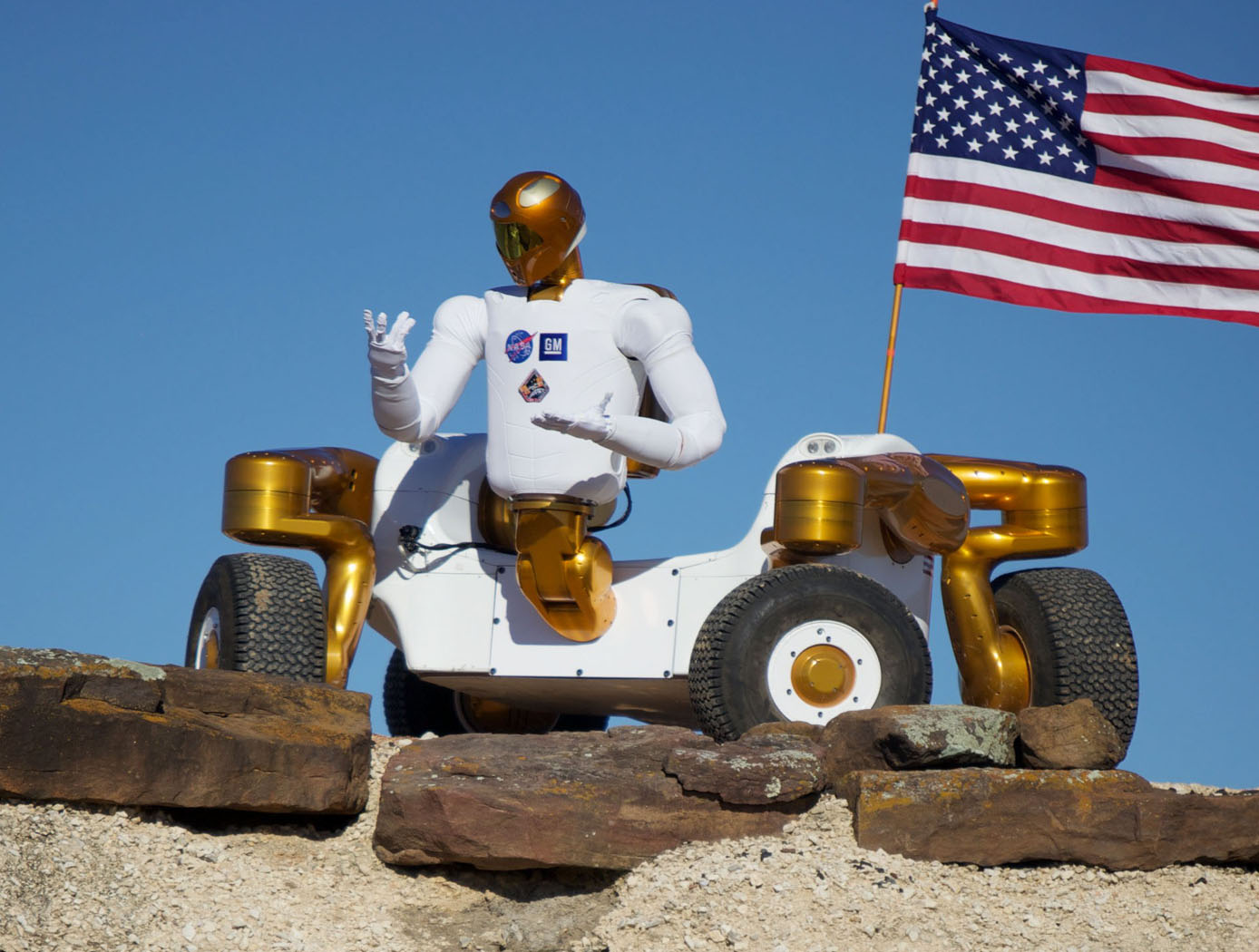
Robonaut 2: Hazardous Environments
Robonaut 2 (R2) has the capability of functioning autonomously or it can be controlled by direct teleoperations, which is advantageous for hazardous environments. When functioning autonomously, R2 understands what to do and how to do it based on sensory input. R2's torso holds the control system while the visor holds several cameras that are incorporated into the visual perception system. With these capabilities, R2 can reduce or eliminate the need for humans to be exposed to dangerous environments. R2 also has a very rugged four-wheel base called the Centaur 2. The Centaur 2 base can lower or raise itself to and from the ground and turn its wheels in any direction, allowing it to turn in place and drive forward or sideways. This enables the R2 to enter hazardous areas or tackle difficult terrain without endangering its human operator.
Robonaut 2 as a whole, or some of its components, can be an invaluable tool for land mine detection, bomb disposal, search and rescue, waste recycling, medical quarantined area, and so much more. The suite of technologies provides an ability to manipulate tools to help with a task, or it can tackle many tasks in a row, where a standard robot may not have the dexterity or sensing capability to get the job done. R2 could pick through nuclear waste, measure toxicity levels, and survey areas too remote or dangerous for human inspection. R2 could deal with improvised explosive devices, detect and dispose of bombs or landmines, and operate equipment that can break through walls or doors.
manufacturing
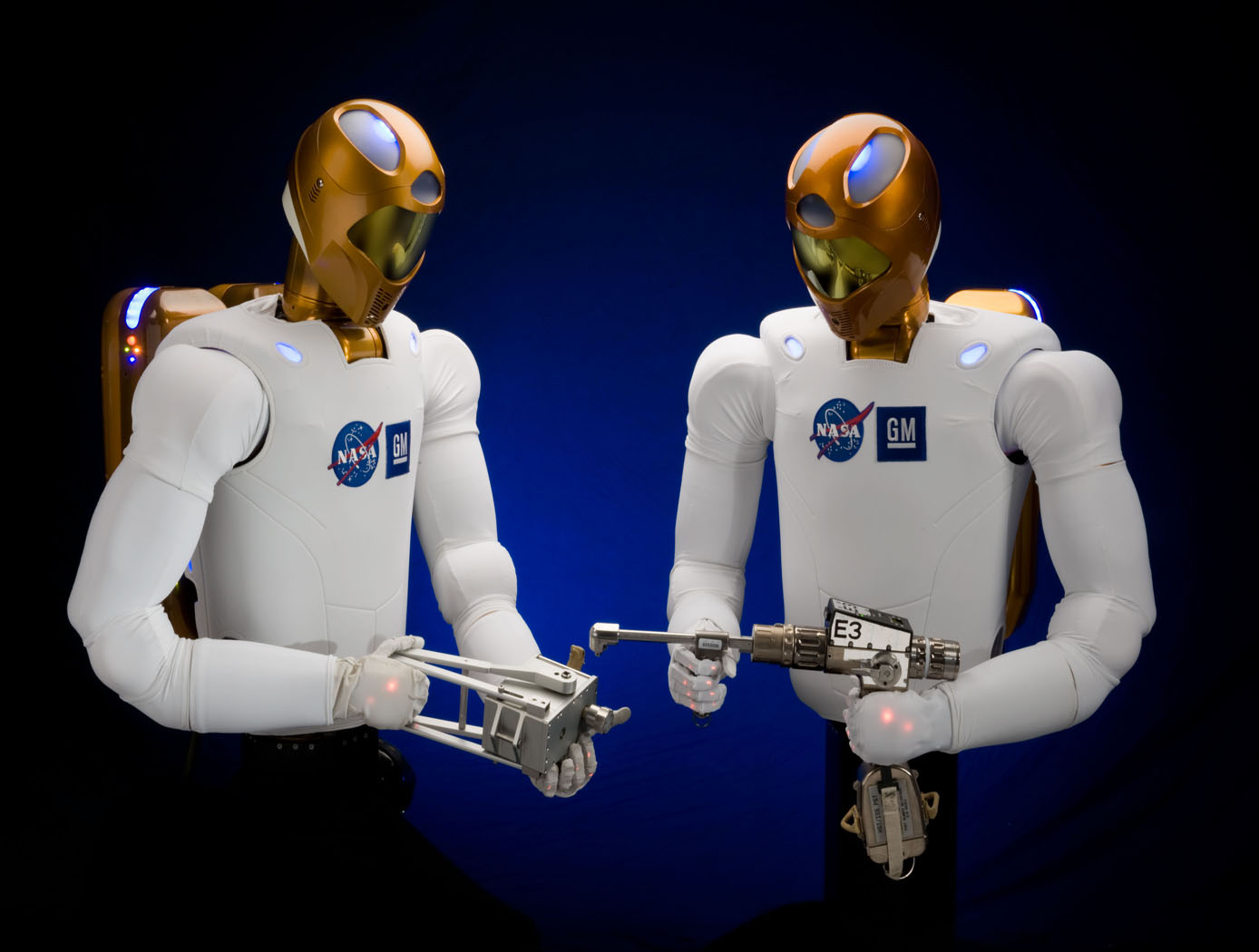
Robonaut 2: Industrial Opportunities
NASA, GM, and Oceaneering approached the development of R2 from a dual use environment for both space and terrestrial application. NASA needed an astronaut assistant able to function in space and GM was looking for a robot that could function in an industrial setting. With this in mind, R2 was made with many capabilities that offer an enormous advantage in industrial environments. For example, the robot has the ability to retool and vary its tasks. Rather than a product moving from station to station on a conveyor with dozens of specialized robots performing unique tasks, R2 can handle several assembly steps at a single station, thereby reducing manufacturing floor space requirements and the need for multiple robots for the same activities. The robot can also be used in scenarios where dangerous chemicals, biological, or even nuclear materials are part of the manufacturing process.
R2 uses stereovision to locate human teammates or tools and a navigation system. The robot was also designed with special torsional springs and position feedback to control fine motor movements in the hands and arms. R2's hands and arms sense weight and pressure and stop when they come in contact with someone or something. These force sensing capabilities make R2 safe to work side-by-side with people on an assembly line, assisting them in ergonomically challenging tasks or working independently.
This NASA Technology is available for your company to license and develop into a commercial product. NASA does not manufacture products for commercial sale.
sensors
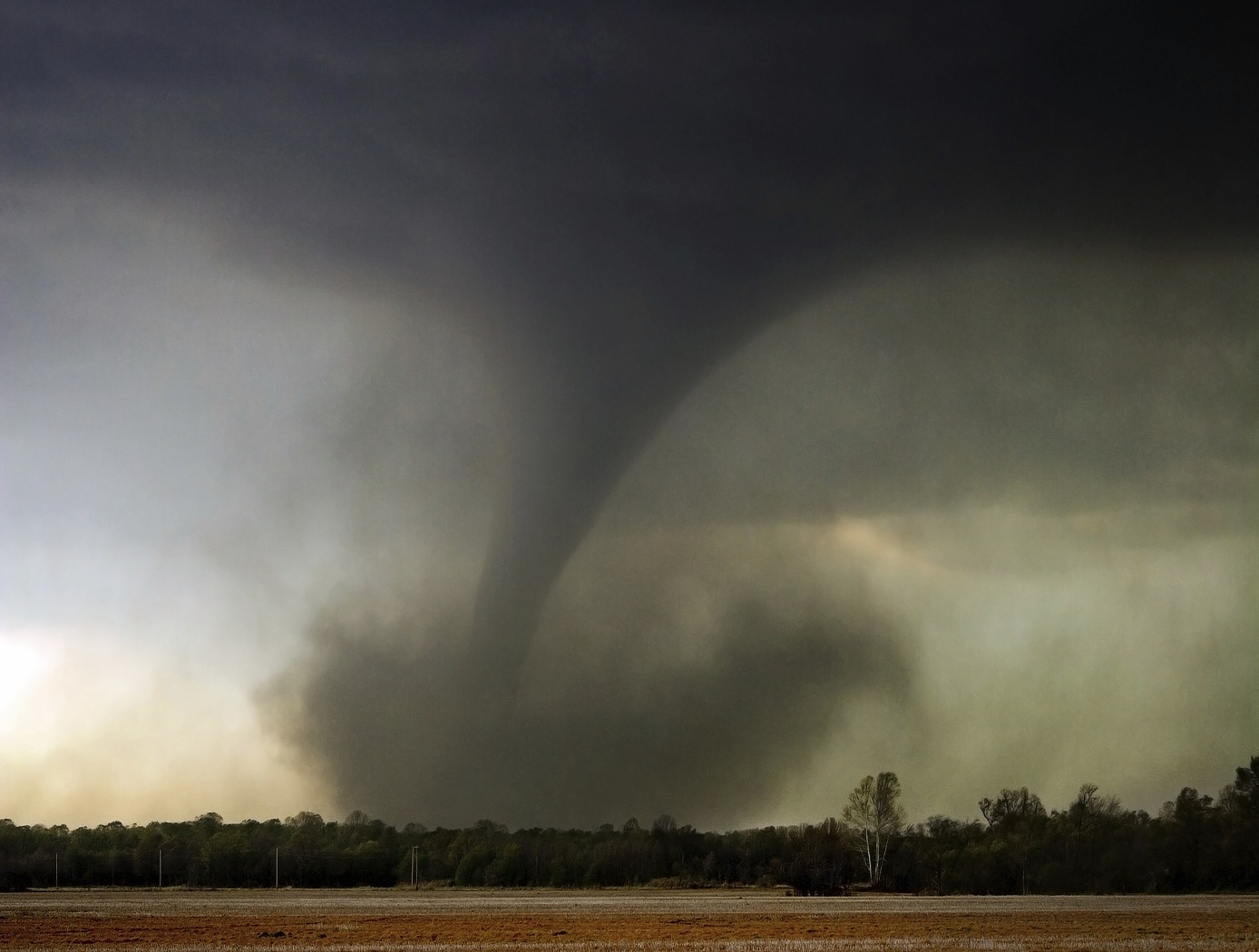
Infrasound Sensor Technology
Large aircraft can generate air vortices in their wake, turbulence that can prove hazardous to aircraft that follow too closely. Because wake vortices are invisible, all takeoffs at busy airports are spaced several minutes apart. This separation gives the vortices time to dissipate, even though they only occur 10% of the time, with resulting loss of operational efficiency. Similarly, clear air turbulence is invisible and can also be hazardous to aircraft. By detecting such disturbances through their infrasound emissions, precautions can be taken to avoid them.
Other phenomena can be detected through infrasound, including tornadoes, helicopters on the other side of mountains, underground nuclear explosions and digging tunnels. Through the unique properties of infrasound, many of these can be detected from hundreds of miles away. NASA's infrasound sensor is a highly refined microphone that is capable of detecting acoustic waves from 20 Hz down to dc, the infrasound range. The design is robust and compact, eliminating the bulk and weight found in other technologies. Where most alternative methods are restricted to certain weather conditions and locations,
the NASA sensor filters noise from wind and other sources, allowing its use under any weather or geographic conditions.
sensors
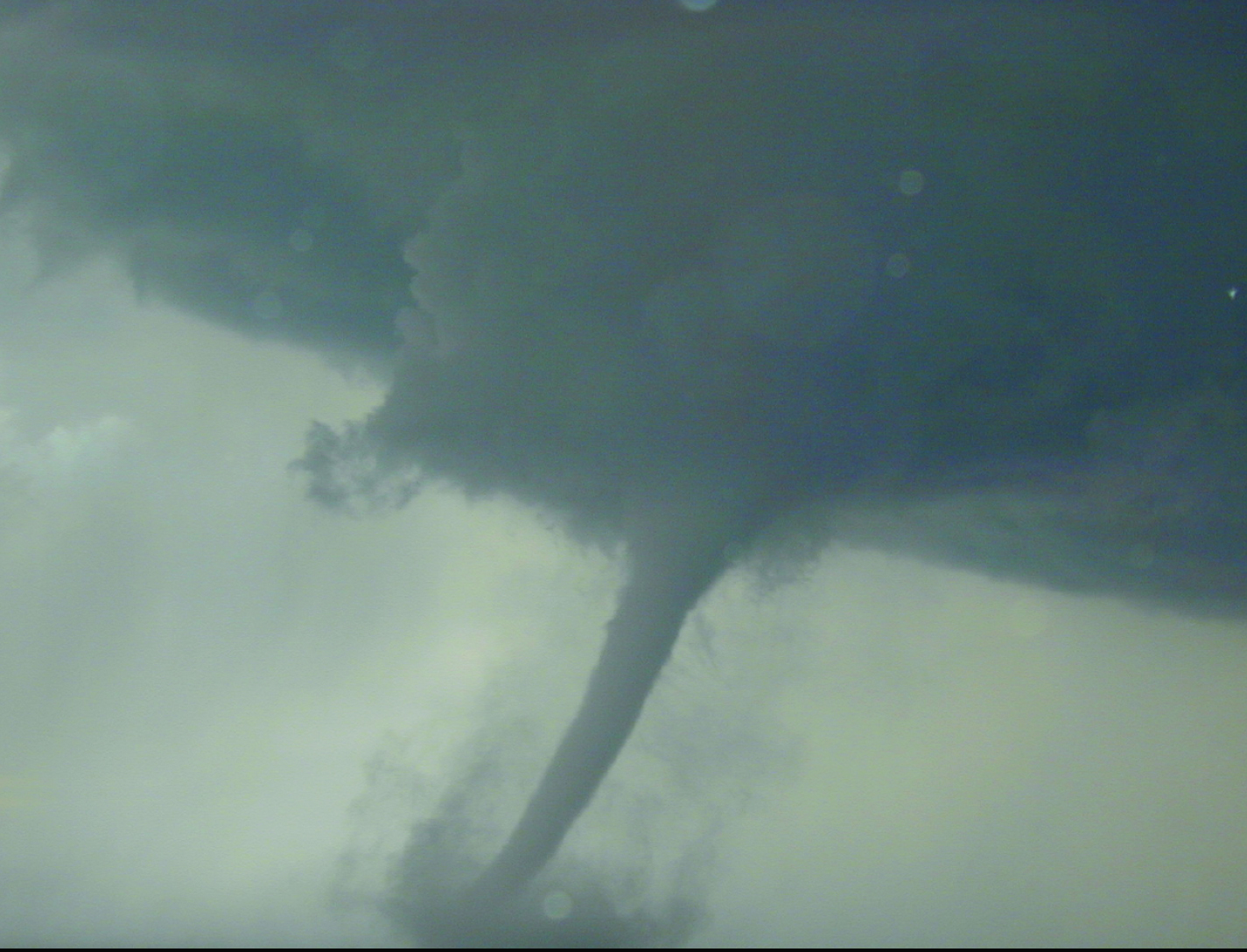
Low Frequency Portable Acoustic Measurement System
Langley has developed various technologies to enable the portable detection system, including:
- 3-inch electret condenser microphone - unprecedented sensitivity of -45 dB/Hz
- compact nonporous windscreen - suitable for replacing spatially demanding soaker hoses in current use
- infrasonic calibrator for field use - piston phone with a test signal of 110 dB at 14Hz.
- laboratory calibration apparatus - to very low frequencies
- vacuum isolation vessel - sufficiently anechoic to permit measurement of background noise in microphones at frequencies down to a few Hz
- mobile source for reference - a Helmholtz resonator that provides pure tone at 19 Hz
The NASA system uses a three-element array in the field to locate sources of infrasound and their direction. This information has been correlated with PIREPs available in real time via the Internet, with 10 examples of good correlation.
mechanical and fluid systems

Additively Manufactured Oscillating Heat Pipe for High Performance Cooling in High Temperature Applications
The advent of additive manufacturing makes available new and innovative integrated thermal management systems, including integrating an oscillating Heat Pipe (OHP) into the leading edge of a hypersonic vehicle for rapid dissipation of large quantities of heat. OHPs have interconnected capillary channels filled with a working fluid that forms a train of liquid plugs and vapor bubbles to facilitate rapid heat transfer. Multiple additive manufacturing techniques may be used, including powder bed fusion, binder jetting, metal material extrusion, directed energy deposit, sheet lamination, ultrasonic, and electrochemical techniques. These high performance OHPs can be made with materials such as Refractory High Entropy Alloys (RHEAs) that can withstand high temperature applications. The structure of the OHP can be integrated into the constructed leading edge. The benefits include a heat transport capacity of 10 to 100 times greater than before. Integrated OHPs avoid the bends or welds in traditional heat pipes, especially at the locations where the highest thermal stresses might cause thermal-structural failure of a leading edge. Alternating the diameters of the OHP channels alleviate start-up issues typically found in liquid metal oscillating heat pipe designs in high temperature applications by aiding in the instigation of a circulating flow due to multiple forces acting upon the working fluid.