Aerospace Vehicle Entry Flightpath Control
aerospace
Aerospace Vehicle Entry Flightpath Control (TOP2-303)
Control System that can be feasibly integrated with a Deployable Entry Vehicle (DEV)
Overview
The state-of-the-art in hypersonic entry Guidance and Control (G&C) is traditionally based on rigid entry vehicles, like the Orion capsule, Space Shuttle, etc., that use a Reaction Control System (RCS) to control the bank angle of the vehicle. However, for future high mass missions to Mars, advancements in hypersonic aerospace vehicle design, and evolving science goals, are stretching the performance capabilities of traditional entry systems.
NASAs Pterodactyl team at Ames Research Center has developed a novel yaw-to-bank control system for a non-traditional vehicle configurations, such as a Deployable Entry Vehicle (DEV) that can be folded and stowed. The approach provides vehicle stabilization, steering, and precise targeted landing. This control architecture is agnostic to control actuators and can be used with any aerospace vehicle with a strong dihedral effect and/or different guidance control variables.
The Technology
This novel flightpath control system exploits the dihedral effect to control the bank angle of the vehicle by modulating sideslip (Figure 1). Exploiting the dihedral effect, in combination with significant aerodynamic forces, enables faster bank accelerations than could be practically achieved through typical control strategies, enhancing vehicle maneuverability. This approach enables vehicle designs with fewer control actuators since roll-specific actuators are not required to regulate bank angle. The proposed control method has been studied with three actuator systems (figure below), Flaps Control System (FCS); Mass Movement Control System (MMCS); and Reaction Control System (RCS).
FCS consists of a flap configuration with longitudinal flaps for independent pitch control, and lateral flaps generating yaw moments. The flaps are mounted to the shoulder of the vehicles deployable rib structure. Additionally, the flaps are commanded and controlled to rotate into or out of the flow. This creates changes in the vehicles aerodynamics to maneuver the vehicle without the use of thrusters.
MMCS consists of moveable masses that are mounted to several ribs of the DEV heatshield, steering the vehicle by shifting the vehicles Center of Mass (CoM). Shifting the vehicles CoM adjusts the moment arms of the forces on the vehicle and changes the pitch and yaw moments to control the vehicles flightpath.
RCS thrusters are mounted to four ribs of the open-back DEV heatshield structure to provide efficient bank angle control of the vehicle by changing the vehicles roll. Combining rib-mounted RCS thrusters with a Deployable Entry Vehicle (DEV) is expected to provide greater downmass capability than a rigid capsule sized for the same launch
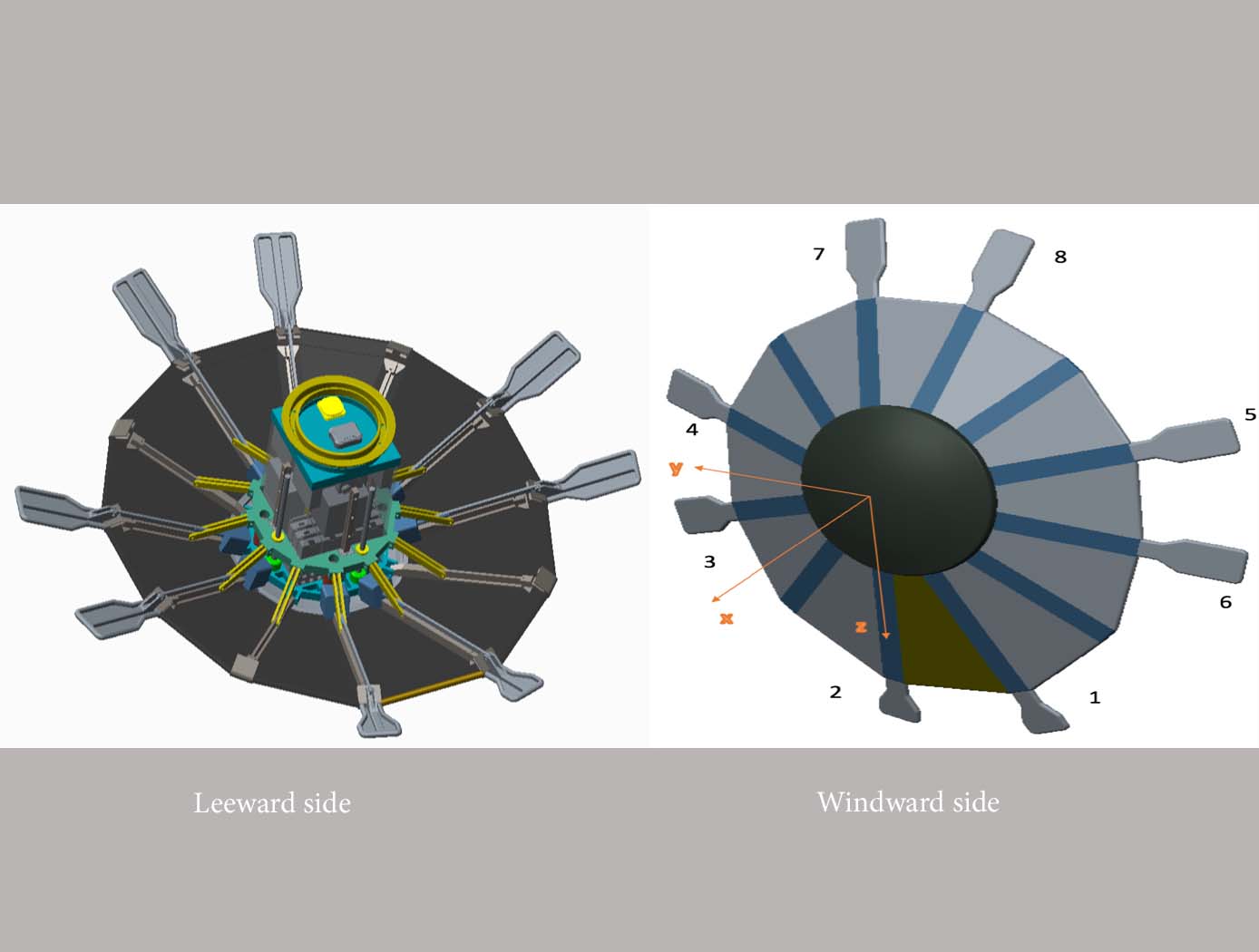
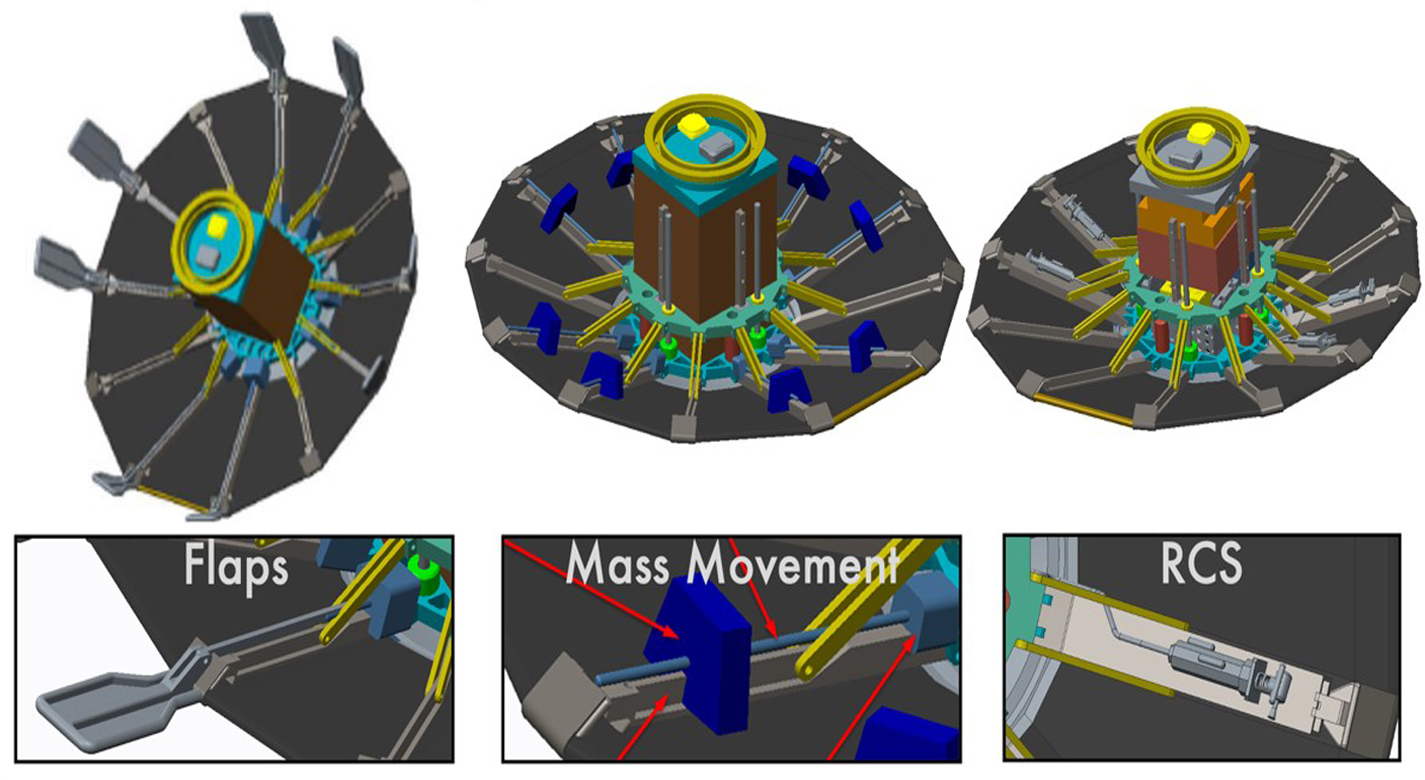
Benefits
- Enables the successful integration of non-propulsive control systems to realize vehicle configuration benefits that include: reductions in propellant mass requirements, net mass savings for the vehicle, and enhanced maneuverability from faster banking accelerations than possible with traditional systems.
- Provides a flexible control architecture applicable to: - aerospace vehicles flying subsonic, supersonic, and hypersonic flight speeds - different types of control actuators (e.g. MMCS, FCS, RCS, etc.)
Applications
- Aerodynamic bodies that require deployable control surfaces on articulating bodies
- Guidance and Control (G&C) of Deployable Entry Vehicles (DEVs) with high precision
- Precision landing of small payloads sent from planetary bodies back to Earth (e.g., biological samples landed near scientific facilities), and (2) increasing down-mass capabilities to planetary bodies such as Mars
- Reentry vehicles and precision space launched or ballistic munitions industry
Technology Details
aerospace
TOP2-303
ARC-18469-1
DSouza, et al., Pterodactyl: System Analysis of an Asymmetric and Symmetric Deployable Entry Vehicle for Precision Targeting Using Flaps, https://doi.org/10.2514/6.2021-0762.
Alunni, et al., Pterodactyl: Trade Study for an Integrated Control System Design of a Mechanically Deployed Entry Vehicle, https://doi.org/10.2514/6.2020-1014.
Okolo, et al., Pterodactyl: Development and Comparison of Control Architectures for a Mechanically Deployed Entry Vehicle, https://doi.org/10.2514/6.2020-1012.
Johnson, et al.,Pterodactyl: Development and Performance of Guidance Algorithms for a Mechanically Deployed Entry Vehicle, https://doi.org/10.2514/6.2020-1011.
Alunni, et al., Pterodactyl: Trade Study for an Integrated Control System Design of a Mechanically Deployed Entry Vehicle, https://doi.org/10.2514/6.2020-1014.
Okolo, et al., Pterodactyl: Development and Comparison of Control Architectures for a Mechanically Deployed Entry Vehicle, https://doi.org/10.2514/6.2020-1012.
Johnson, et al.,Pterodactyl: Development and Performance of Guidance Algorithms for a Mechanically Deployed Entry Vehicle, https://doi.org/10.2514/6.2020-1011.